What is Ethereum? The Ultimate Guide to Understanding ETH
The article provides an ultimate guide to understanding Ethereum, highlighting its key features, use cases, and advantages. It starts by emphasizing the increasing interest in Ethereum compared to Bitcoin, particularly among institutional investors.
The article then introduces Ethereum as a decentralized network that uses blockchain technology to run applications and process transactions. It explains that Ethereum has its own cryptocurrency called Ether (ETH), which is used to power the network and pay for network fees. It also highlights that Ethereum enables the creation of decentralized applications (DApps) through smart contracts, which are self-executing agreements that enforce rules and conditions without the need for intermediaries.
The article further explores Ethereum's key features, including its decentralized application ecosystem, where users can build and run various applications, such as finance, gaming, and social media. It also mentions decentralized autonomous organizations (DAOs), which are organizations governed by their members through voting on proposals using smart contracts. Additionally, the article introduces the Ethereum Virtual Machine (EVM), which allows any code compatible with EVM to run and perform computations on the Ethereum network.
The article then delves into the technical aspects of Ethereum, explaining how the network operates with nodes, validators, stakers, and developers. It covers concepts like gas, nonce, gas fees, and Ethereum's block size limit. It highlights the differences between Ethereum and Bitcoin, such as their consensus mechanisms (Proof of Stake for Ethereum and Proof of Work for Bitcoin) and block times.
Next, the article explores Ethereum's use cases, including decentralized finance (DeFi), non-fungible tokens (NFTs), and decentralized autonomous organizations (DAOs). It explains how Ethereum facilitates peertopeer lending, the trading of digital assets, and the creation of decentralized organizations. It also mentions Ethereum's role as a data availability and settlement layer.
The article concludes by discussing Ethereum's advantages, such as its first-mover advantage, composability, network security, and robustness. It reaffirms Ethereum's future potential by outlining upcoming developments, including the Shapella and Verge upgrades, which will enhance scalability and transaction processing speed.
Overall, the article provides a comprehensive and detailed overview of Ethereum, explaining its purpose, functionality, and potential impact on various industries. It offers valuable insights for both newcomers and those familiar with the cryptocurrency and blockchain space.
The article provides an ultimate guide to understanding Ethereum, highlighting its key features, use cases, and advantages. It starts by emphasizing the increasing interest in Ethereum compared to Bitcoin, particularly among institutional investors.
The article then introduces Ethereum as a decentralized network that uses blockchain technology to run applications and process transactions. It explains that Ethereum has its own cryptocurrency called Ether (ETH), which is used to power the network and pay for network fees. It also highlights that Ethereum enables the creation of decentralized applications (DApps) through smart contracts, which are self-executing agreements that enforce rules and conditions without the need for intermediaries.
The article further explores Ethereum's key features, including its decentralized application ecosystem, where users can build and run various applications, such as finance, gaming, and social media. It also mentions decentralized autonomous organizations (DAOs), which are organizations governed by their members through voting on proposals using smart contracts. Additionally, the article introduces the Ethereum Virtual Machine (EVM), which allows any code compatible with EVM to run and perform computations on the Ethereum network.
The article then delves into the technical aspects of Ethereum, explaining how the network operates with nodes, validators, stakers, and developers. It covers concepts like gas, nonce, gas fees, and Ethereum's block size limit. It highlights the differences between Ethereum and Bitcoin, such as their consensus mechanisms (Proof of Stake for Ethereum and Proof of Work for Bitcoin) and block times.
Next, the article explores Ethereum's use cases, including decentralized finance (DeFi), non-fungible tokens (NFTs), and decentralized autonomous organizations (DAOs). It explains how Ethereum facilitates peertopeer lending, the trading of digital assets, and the creation of decentralized organizations. It also mentions Ethereum's role as a data availability and settlement layer.
The article concludes by discussing Ethereum's advantages, such as its first-mover advantage, composability, network security, and robustness. It reaffirms Ethereum's future potential by outlining upcoming developments, including the Shapella and Verge upgrades, which will enhance scalability and transaction processing speed.
Overall, the article provides a comprehensive and detailed overview of Ethereum, explaining its purpose, functionality, and potential impact on various industries. It offers valuable insights for both newcomers and those familiar with the cryptocurrency and blockchain space.
If you're starting to explore blockchain technology, chances are the first two names you'll come across are Bitcoin and Ethereum. Together, these two giants hold the majority of value in the blockchain industry.
Bitcoin, as the most valuable asset in the space, is built to protect that status. Its network functions like SWIFT, providing a peer-to-peer system for transferring Bitcoin and maintaining an unchangeable record of BTC transactions. But while Bitcoin focuses on being a digital asset, Ethereum is built to be much more than just a token transfer system.
A helpful way to think about Ethereum is to imagine it as a global computer—similar to the one you're using right now. Ethereum's protocol allows users to run programs and perform various operations, offering a platform for decentralized applications (DApps) and smart contracts. In fact, Ethereum's innovation in this area laid the foundation for the rise of Decentralized Finance (DeFi), Real World Assets (RWAs), Decentralized Physical Infrastructure Networks (DePINs), Non-Fungible Tokens, and just about every other use case you can imagine within Web3 today.
Coin Bureau reviewed Ethereum back in 2021. Since then, the network has evolved through major updates that have significantly shifted its capabilities. That’s why, we’re revisiting Ethereum to ensure the blockchain community, especially newcomers, has a full understanding of what Ethereum represents today.
What is Ethereum?
Having a basic understanding of blockchain technology and the Bitcoin network will be highly beneficial as we delve into the concepts surrounding Ethereum. This foundational knowledge will help you grasp how Ethereum builds upon and differentiates itself from earlier blockchain technologies like Bitcoin.
At its most fundamental level, Ethereum is a blockchain network. It operates as a public database that is a vast network of computers, known as nodes, that updates and maintains collectively in real time. Ethereum periodically records and updates this database's state in units called blocks, each referencing its predecessor to form a continuous chain known as the blockchain. To ensure all nodes agree upon the state of the blockchain, Ethereum employs a consensus mechanism called Proof of Stake (PoS).
Broad Use Cases of the Ethereum Network
A Peer-to-Peer Network and Decentralized Ledger
The Ethereum network features Ether (ETH) as its native cryptocurrency. Similar to how Bitcoin operates, Ethereum enables peer-to-peer (P2P) transfers of Ether, allowing users to send and receive value without intermediaries. The primary role of the Ethereum protocol in this context is to facilitate these P2P transactions and maintain the network by compensating nodes—validators—with ETH through its monetary policy. Ether's utility within Ethereum mirrors Bitcoin's utility within its own network, serving as both a medium of exchange and a reward for those who help secure the network.
A Decentralized, Censorship-Resistant Computer
Beyond simple value transfers, the Ethereum network functions as a single, canonical computer known as the Ethereum Virtual Machine (EVM). Nodes can request the EVM to perform arbitrary computations, which every participating node executes to verify the computation's correctness. The blocks contain information about the operations performed during their period, and the consensus process ensures every node has the same copy of the results, known as the common block state.
These computation requests consume resources and are paid for using a metered system called gas. Gas fees are measured and paid in denominations of Ether. This mechanism ensures that the network remains efficient and prevents abuse of computational resources. Importantly, the entire operation is censorship-resistant; as long as you have an internet connection, no one can prevent you from interacting with the network. Cryptographic mechanisms ensure that once transactions are verified as valid and added to the blockchain, they cannot be altered or tampered with later.
A Source of Credible Block Space
While Ethereum has always provided block space for transactions and computations, it has recently emerged as a monetized resource within the ecosystem. Ethereum's block space is considered one of the most credible, decentralized, and secure chunks of data space available, second only to Bitcoin. This credibility stems from Ethereum's extensive validator network and its substantial monetary resources, collectively guaranteeing its security. The scarcity of block space also ensures its optimal use.
Other blockchain networks can leverage Ethereum's credible block space to run their operations in a sandbox environment within the Ethereum network. This allows them to benefit from Ethereum's security and decentralization without building and securing their own networks from scratch.
Ethereum's Use Cases
These use cases highlight the transformative potential of Ethereum, enabling:
- Censorship-Resistant Networking and Money: Users can transact and interact without fear of interference or censorship from central authorities.
- An Open Internet: Ethereum supports the development of decentralized applications (DApps), fostering an Internet where data and services are open and accessible.
- A Base for Decentralized Finance (DeFi): Ethereum's smart contract capabilities underpin various financial services that operate without traditional intermediaries.
- Composable Online Products: Developers can build modular applications that interoperate seamlessly, encouraging innovation and collaboration within the ecosystem.
In essence, Ethereum is more than just a cryptocurrency; it's a versatile platform that empowers users and developers to create and participate in a decentralized digital economy.
Fundamental Concepts in Ethereum Protocol
Here are some foundational topics about the Ethereum protocol that will help build essential context for more complex discussions further into the piece:
Ether (ETH)
Ether (ETH) serves as the native cryptocurrency of the Ethereum protocol, much like Bitcoin (BTC) does for the Bitcoin network. However, Ether's purpose extends beyond being a store of value; it powers an ecosystem of decentralized applications, smart contracts, and financial instruments.
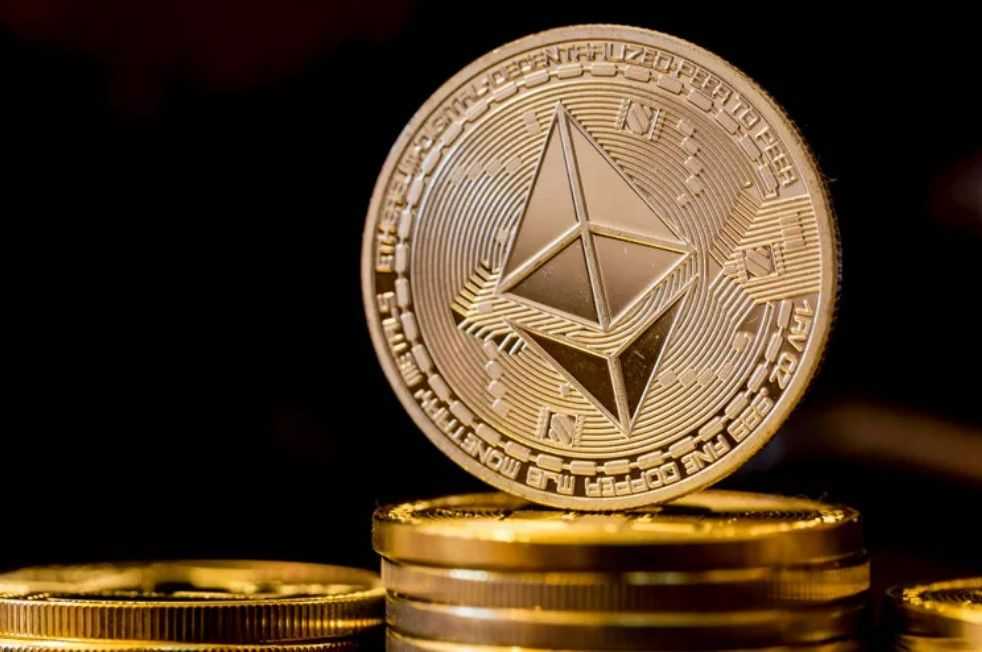
Ether and Bitcoin: Similar Foundations, Different Purposes
Just as Bitcoin maintains a canonical and immutable ledger of BTC transactions, Ethereum does the same for Ether. "Canonicity" refers to the idea that the blockchain's record of Ether transactions is the one true record, accepted across the entire network. Once a transaction is confirmed, it becomes part of Ethereum’s immutable ledger, meaning it cannot be altered or erased. This immutability is crucial, as it guarantees trust in the system, ensuring that no one can tamper with Ether transactions or manipulate balances.
For example, imagine a shared document where changes can only be added but never deleted or edited; everyone reading the document can see the complete history. This quality makes Ether transactions transparent and resistant to fraud, forming the backbone of trust in Ethereum’s ecosystem.
Ether as the Medium of Exchange within Ethereum
On Ethereum, Ether is more than just a currency—it’s also a network exchange medium. To understand this, imagine Ethereum as a global computer, the Ethereum Virtual Machine (EVM), which processes smart contracts programs. Running these programs requires computational power, and just like running any machine, it costs a fee, known as gas.
Gas fees, which measure the computational work required for transactions, are paid to validators in Ether. These validators, the participants who verify and secure transactions on the network, earn ETH in return for their work. For example, if you initiate a transaction or interact with a smart contract, a small amount of Ether is paid as gas, compensating validators and ensuring the network runs smoothly.
Ether’s Monetary Policy: Inflation and Burning
Ethereum’s monetary policy includes both inflationary and deflationary elements. When a new block is created, new units of Ether are minted, contributing to a controlled inflation rate. However, Ether also has a mechanism to counterbalance inflation—transaction fees, or a portion of gas fees, are "burned" or permanently removed from circulation.
"Burning" is a process where a specific amount of Ether is destroyed by sending it to an address without a private key. Depending on the volume of network activity, this burning mechanism can reduce the total supply of Ether over time. We’ll explore this burning mechanism further in a later section, where we’ll cover its long-term impact on Ethereum’s economy.
Ether as a Tool for Network Security
Securing the Ethereum network requires participants to pledge some of their Ether as a stake in the network, which acts as a deterrent against malicious behavior. Validators must lock up a certain amount of Ether in a process called staking. By doing so, they have a vested interest in the network’s success and integrity, as they risk losing their staked Ether if they act dishonestly or attempt to disrupt the network.
Think of it as a deposit in a high-stakes game; participants risk losing their deposit if they play unfairly. This staking model is fundamental to Ethereum’s Proof of Stake (PoS) consensus, which we’ll explore in more detail later.
Denominations of Ether: Wei and Gwei
Ether can be divided into smaller units, allowing for precise transactions even when dealing with fractions of ETH. Two common denominations are:
- Wei: The smallest unit of Ether, equivalent to one quintillionth (10^-18) of an ETH. If we compare Ether to a dollar, Wei would be like a single cent but on a much smaller scale.
- Gwei: Often used for gas fees, Gwei represents one billion Wei or 10^-9 ETH. When you see gas prices quoted, they’re typically expressed in Gwei for convenience. For instance, if the gas fee for a transaction is 20 Gwei, it’s actually referring to 20 billion Wei.
These denominations make it possible to manage even very small values efficiently, a practical feature given Ethereum’s role in executing high-volume, low-cost transactions.
Ethereum Accounts
Private Keys and Public Keys: The Cryptographic Foundation
At the heart of every Ethereum account lies a private and public key, which are essential to secure transactions. These keys are created using the Elliptic Curve Digital Signature Algorithm (ECDSA) cryptography, specifically the secp256k1 curve. Here’s a simplified view of how it works:
- Private Key: This is a randomly generated 256-bit number, which serves as a unique digital signature. A private key is kept secret and must never be shared.
Example of a private key: 0x9c1c77ab91d10d71012d61dcb7b12aef8d509a2d13ed7c54f1e347b89720dc40 - Public Key: The private key is mathematically transformed using ECDSA to create a corresponding public key. The ECDSA transformation process makes it practically impossible to reverse engineer the private key from the public key, which is crucial for security.
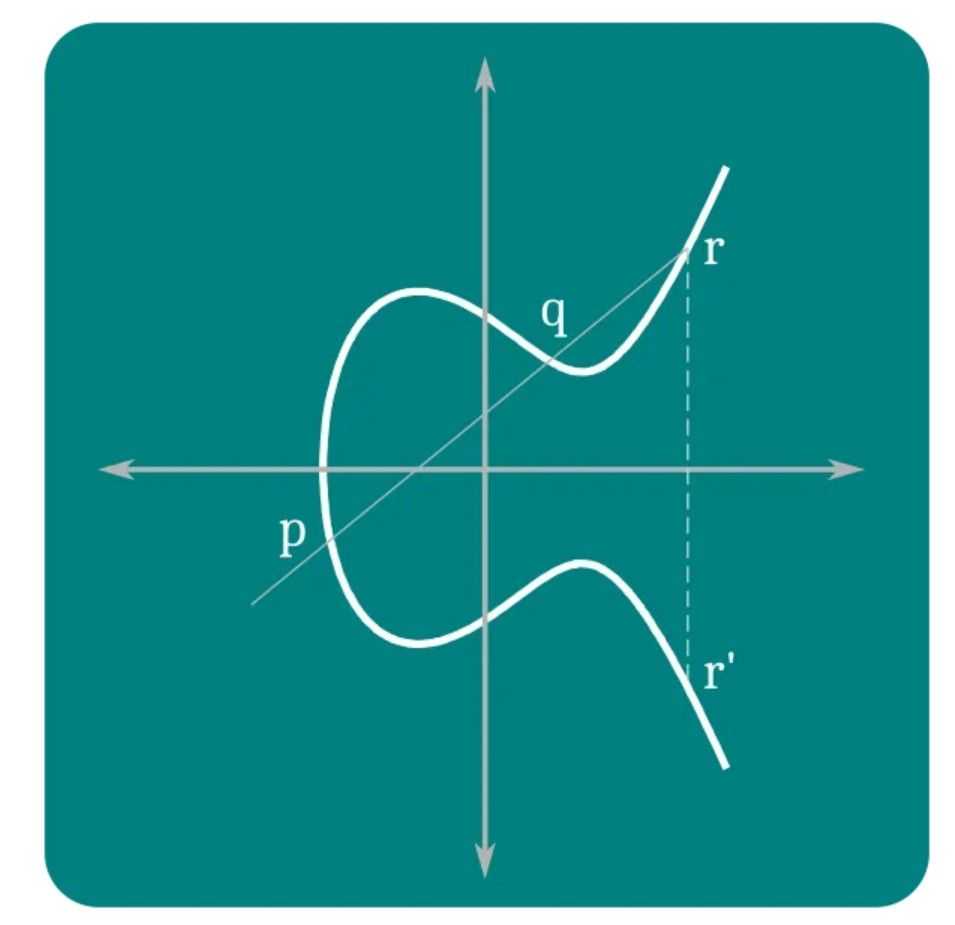
Because the cryptographic algorithms used are one-way functions (known as hashing), public keys can be freely shared without exposing the private key. To put it in perspective, reversing a private key from a public key would require more computational power than exists in the world, making Ethereum accounts secure.
Externally Owned Accounts (EOAs) and Key Pairs
Most users interact with Ethereum using Externally Owned Accounts (EOAs), which rely on key pairs. Each EOA is controlled by a private key, which provides exclusive access to the account’s funds and transaction abilities. Along with the private key, an EOA has a public address—a simplified form of the public key that represents the account.
A public address is essentially the public face of an account, where others can send Ether or tokens. For example, if Alice wants to send Bob some Ether, she would use Bob’s public address as the destination. The private key enables Bob to authorize transactions from his account, ensuring he can only spend his funds.
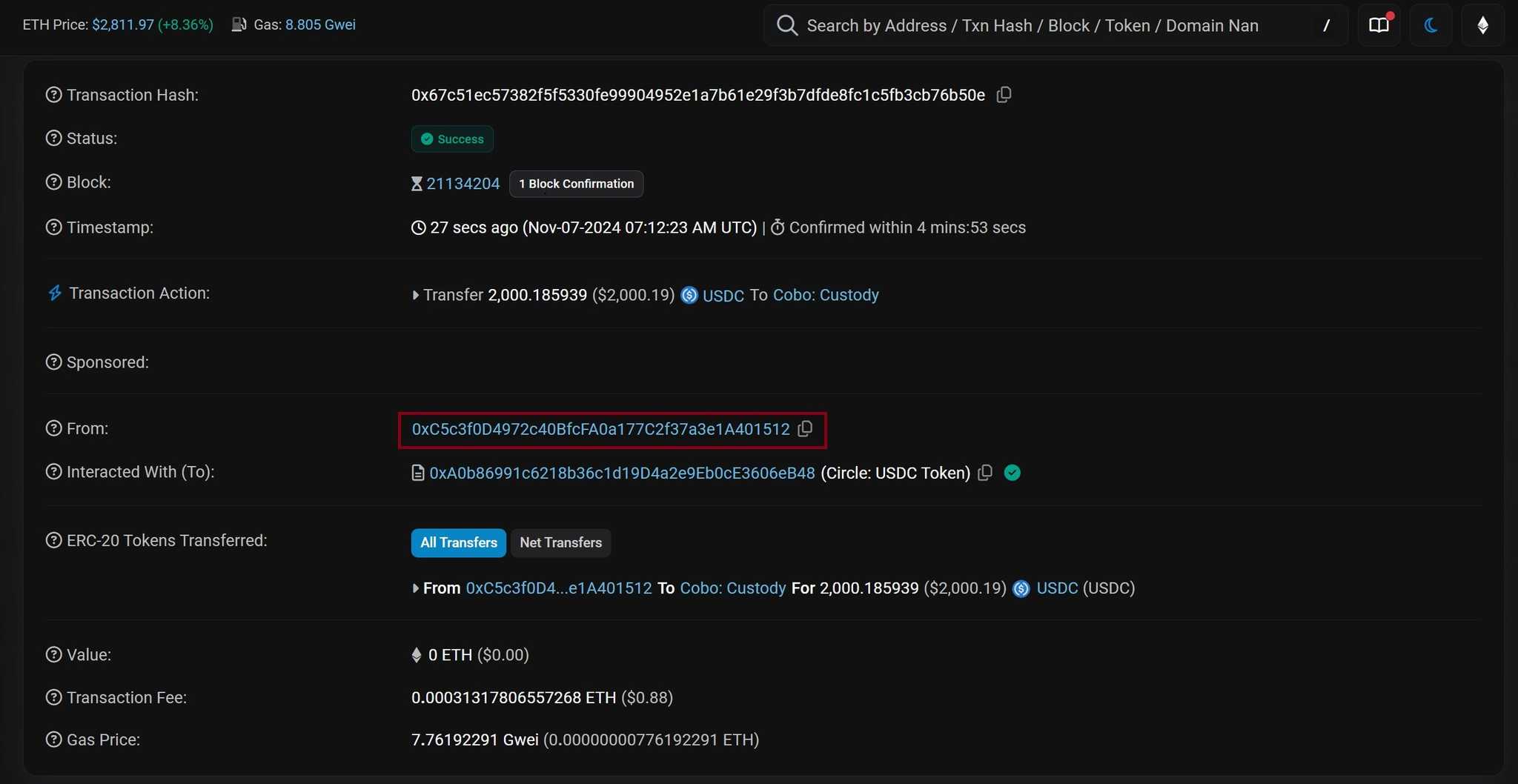
Wallets: A Practical Interface for EOAs
A wallet is a software or hardware interface that allows users to interact with Ethereum and manage their EOAs. Wallets store and manage the private and public keys, enabling users to send, receive, and view their assets without handling raw cryptographic details.
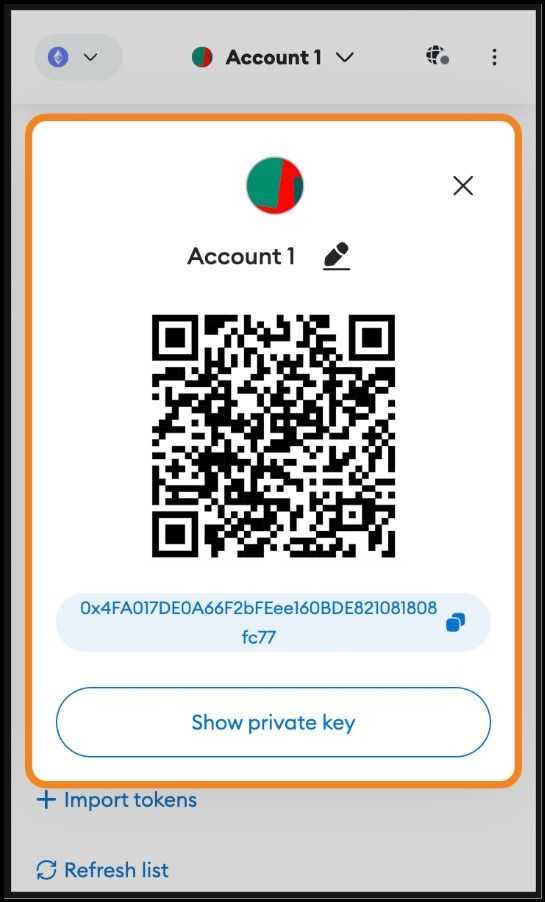
Hot Wallets and Cold Wallets
There are two primary types of wallets:
- Hot Wallets: These are connected to the internet, making them convenient for frequent transactions. Examples of hot wallet providers include MetaMask and Trust Wallet. However, hot wallets are more susceptible to online attacks, so they are generally recommended for smaller amounts.
- Cold Wallets: These are offline wallets, which store private keys on hardware devices or paper, providing enhanced security. Popular cold wallets include Ledger and Trezor, ideal for long-term storage of substantial funds since they are offline and therefore more resistant to hacking.
Learn deeper insights into what a wallet address is, or check out Coin Bureau’s list of the Best Ethereum Wallets!
Contract Accounts: Extending Ethereum’s Utility
Beyond EOAs, Ethereum has contract accounts. Unlike EOAs, contract accounts do not have private keys; instead, they are controlled by code called smart contracts.
- Creation and Function: Contract accounts are created when users deploy smart contracts to the Ethereum blockchain. Once created, these accounts operate independently, following predefined rules encoded in the contract.
- Limitations: Contract accounts cannot initiate transactions independently—they must be triggered by an EOA. This design ensures that contract accounts only act when external input is provided, preventing unauthorized actions.
Smart Contracts: The Core of Contract Accounts
Smart contracts are essentially programs deployed on the Ethereum blockchain. When a smart contract is deployed, it becomes a contract account with a unique address, enabling others to interact with it. For example, a decentralized exchange (DEX) contract account could execute trades automatically based on the conditions set in its code.
Because contract accounts are controlled by code, they have specific functions and limitations. They cannot hold private keys or initiate transactions, meaning all operations must be triggered externally, often by EOAs.
Triggering Smart Contracts: Example of an EOA-Contract Interaction
Imagine Alice wants to swap Ether for a token using a DEX smart contract. Here’s how this interaction unfolds:
- Alice’s EOA initiates the transaction by sending a message to the DEX’s contract account, specifying the swap details, and attaching the required Ether.
- The DEX contract account receives Alice’s request, executes the predefined swap function, and sends back the token to Alice’s account.
- State Changes and Final Block State: This transaction updates the balance of Ether and tokens in Alice’s account and the DEX’s contract account, finalizing the blockchain’s state.
Each step in this process requires computational resources, known as gas. Gas is spent processing the contract code and updating the network’s state. The more complex the operation, the more gas is required, which Alice pays in Ether as compensation for validators.
Solidity: The Language of Smart Contracts
Smart contracts on Ethereum are written in Solidity, a programming language designed specifically for the platform. Solidity allows developers to write code determining how a contract account will behave, defining functions, storage, and rules.
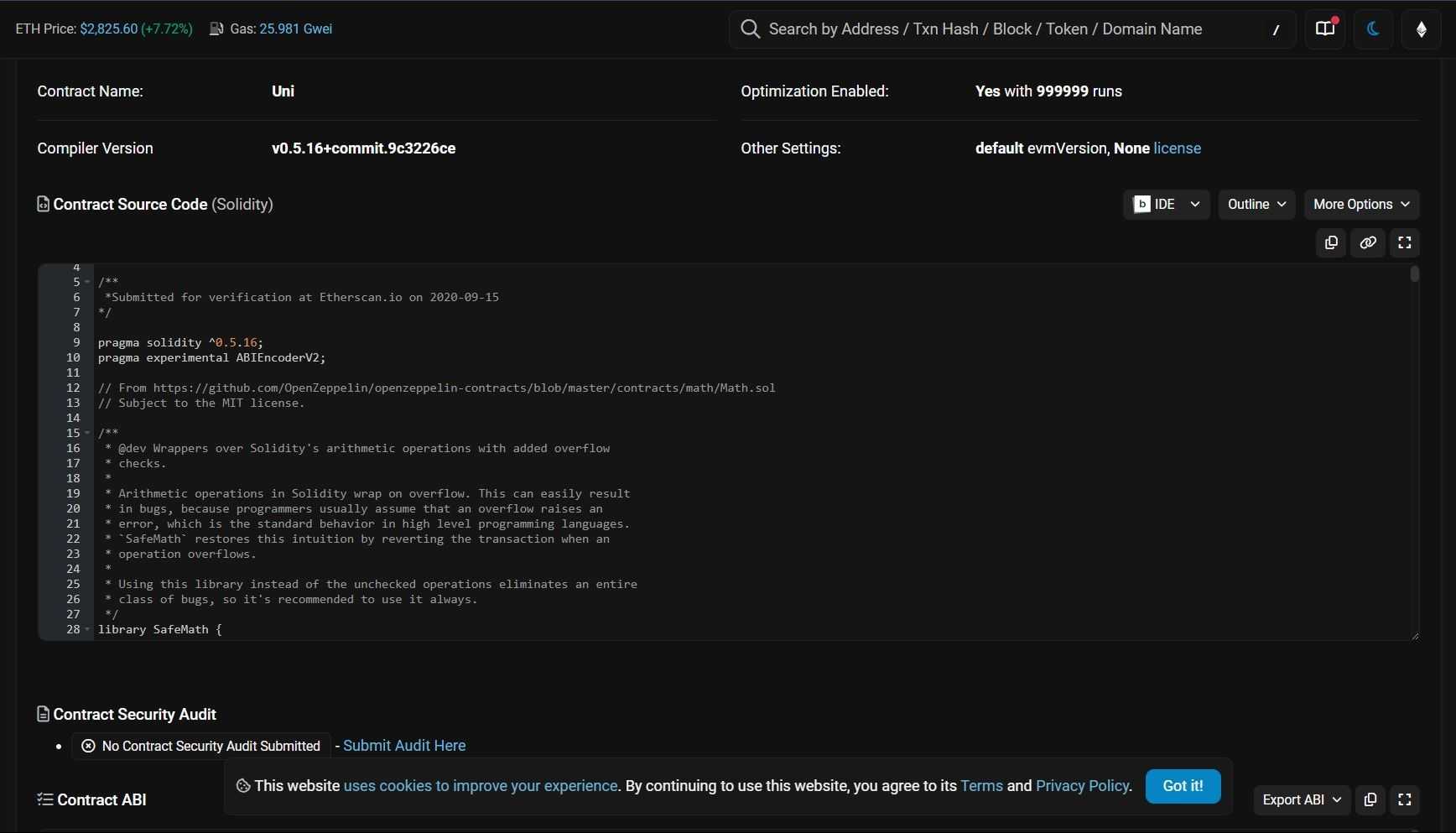
For instance, a crowdfunding contract written in Solidity might include functions to accept funds, check funding goals, and return funds if the target isn’t met. Solidity's syntax is similar to JavaScript, making it accessible to many developers. With Solidity, the Ethereum network has become a flexible platform for developers to create decentralized applications (DApps) across industries.
Ethereum Token Standards: Enabling Versatile Digital Assets
Ethereum has given rise to various token standards, each designed to support different digital assets and applications. Token standards are like templates that define the rules for creating and managing tokens on the Ethereum network. Let’s look at the most popular standards and how they’re used.
ERC-20 Tokens: The Standard for Fungible Tokens
ERC-20 is the most common token standard on Ethereum and is used to create fungible tokens—identical and interchangeable tokens. Each ERC-20 token operates on the same basic principles, making them easy to transfer, store, and exchange across wallets and platforms that support the standard. ERC-20 tokens follow a set of standardized functions, such as transfer and approve, ensuring compatibility across various Ethereum-based applications.
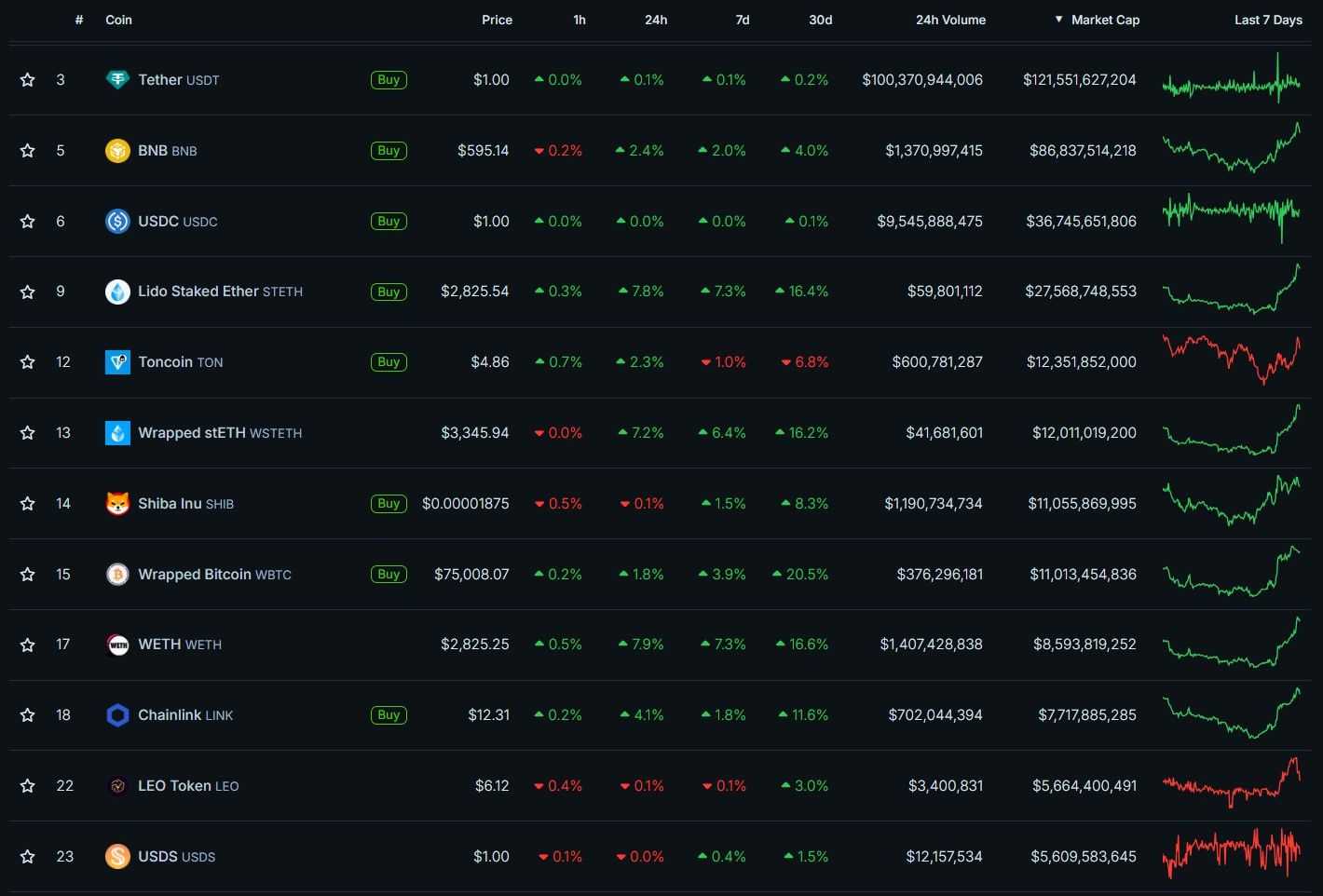
Some well-known examples of ERC-20 tokens include:
- USDT (Tether): A stablecoin pegged to the U.S. dollar, used widely for trading and payments.
- LINK (Chainlink): A token for the Chainlink network, supporting decentralized oracles.
- UNI (Uniswap): A governance token for the Uniswap decentralized exchange.
ERC-20 tokens have become the backbone of decentralized finance (DeFi), powering trading, lending, and staking applications on Ethereum.
ERC-721 Tokens: The Non-Fungible Token Standard
The ERC-721 standard is designed for non-fungible tokens (NFTs)—unique digital assets that cannot be interchanged one-to-one like ERC-20 tokens. Each ERC-721 token has a unique identifier, allowing it to represent individual ownership of a specific asset. This standard has been pivotal in the rise of digital collectibles, artwork, and other assets that benefit from unique, verifiable blockchain ownership. There are dedicated markets where you can trade NFTs. Here is a list of the top NFT Marketplaces.
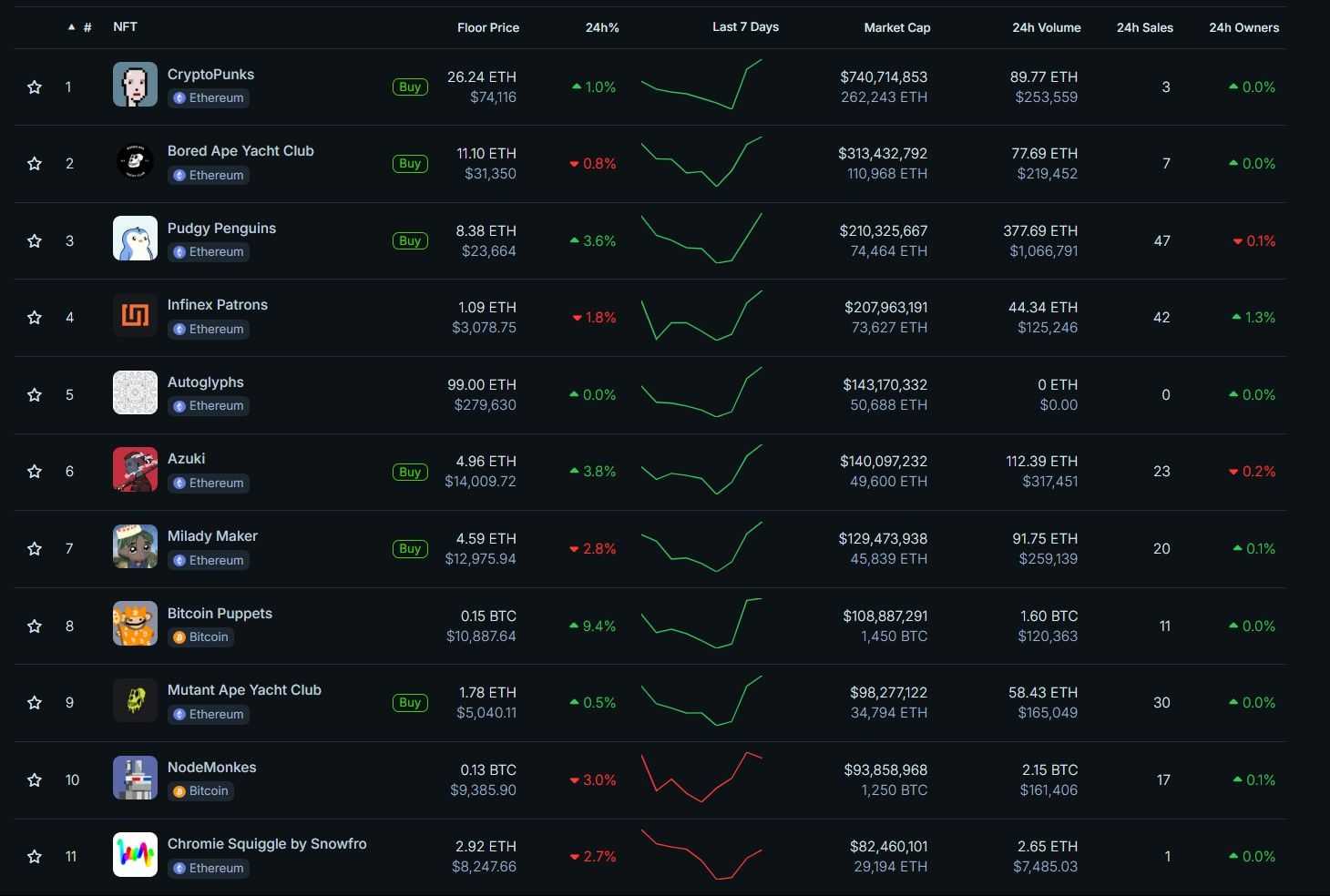
Examples of popular ERC-721 NFTs include:
- CryptoKitties: One of the earliest NFT projects, where users breed and trade digital cats, each with unique characteristics.
- Bored Ape Yacht Club: A collection of distinctive, hand-drawn ape characters that have become highly collectible.
- Decentraland LAND: Virtual real estate parcels in the Decentraland metaverse, each with its own coordinates and unique properties.
ERC-721 tokens have brought a new level of individuality and ownership to digital assets, enabling new forms of value in art, gaming, and virtual real estate.
ERC-1155 Tokens: The Multi-Token Standard
ERC-1155 combines features of both ERC-20 and ERC-721, making it ideal for creating collections that contain fungible and non-fungible tokens. This standard was developed for gaming and other use cases where users might need multiple types of assets within a single contract. For example, in a video game, a player might hold both in-game currency (fungible) and unique weapons or armor (non-fungible), all managed through a single ERC-1155 contract.
Examples of ERC-1155 tokens include:
- Enjin Coin (ENJ): A gaming platform that allows users to create fungible and non-fungible game assets.
- Gods Unchained: A blockchain-based card game where players own unique cards (non-fungible) alongside in-game assets like tokens (fungible).
By allowing multiple assets in a single contract, ERC-1155 reduces the required contracts, making transactions more efficient and less costly.
Tokens as Token Contracts
Tokens on Ethereum are actually token contracts, which are specific types of smart contracts. Each token is a separate contract that maintains its own ledger of transactions and balances. For example, when you buy an ERC-20 token, you aren’t directly holding it in your wallet; instead, the token contract updates its ledger to reflect your ownership. In this way, tokens are special types of smart contracts that operate as decentralized databases for tracking token balances.
When you transfer an ERC-20 token, the token contract updates its internal ledger to decrease your balance and increase the recipient’s. This approach ensures all token-related transactions on the blockchain are transparent and verifiable.
Is Ether an ERC-20 Token? Clearing Up a Common Misconception
One common misconception is that Ether (ETH) is an ERC-20 token. However, this isn’t the case. The ERC-20 standard allowed developers to design new tokens on Ethereum’s network. On the other hand, Ether is Ethereum’s native currency, and Ethereum natively tracks Ether transactions. This makes Ether unique—it doesn’t need a smart contract to manage transactions, as the Ethereum protocol itself does.
Because Ether exists outside of any token contract, it is not an ERC-20 token. Instead, ERC-20 tokens are simply additional assets created on Ethereum using smart contracts, whereas Ether is the currency that fuels the entire Ethereum ecosystem.
Ethereum Blocks: The Building Blocks of the Blockchain
Ethereum blocks are fundamental to the structure of the Ethereum blockchain. They contain batches of transactions, essential data, and references to previous blocks, creating a continuous and verifiable information chain. Let’s explore what Ethereum blocks are, how they’re made, and the components they contain.
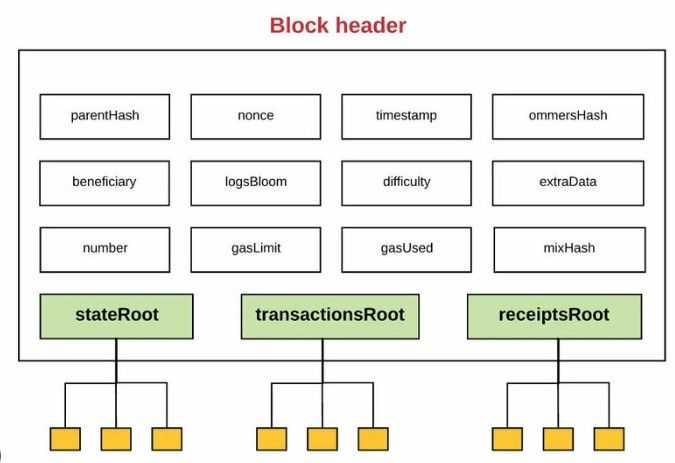
Blocks as Batches of Transactions
An Ethereum block is essentially a bundle of transactions grouped and confirmed together. Each block contains a hash of the previous block, which links it to the chain, forming an unbroken sequence back to the very first block (the genesis block). This structure ensures that every block is connected, making it impossible to modify a single block without altering the entire chain.
This hash-based linkage forms the core of Ethereum’s security and transparency. Because each block depends on the previous one, any attempt to tamper with a past transaction would be immediately apparent. Each participant on the network can verify the entire history of transactions, ensuring that all nodes in the network agree on Ethereum’s precise transaction history and state.
Ensuring Synchronization and Consensus
Blocks are the mechanism by which all network participants maintain a synchronized state of Ethereum. When a new block is added, it updates the entire network with the latest set of confirmed transactions. This synchronization is crucial, as it ensures every node on Ethereum shares a consistent view of account balances, smart contract states, and transaction history.
Through this process, Ethereum’s blockchain achieves consensus—agreement among all nodes in the network’s current state. This consensus is essential for maintaining trust and preventing fraudulent or conflicting records.
Creating a Block: The Role of Validators
In Ethereum’s Proof of Stake (PoS) system, validators create new blocks. Rather than using energy-intensive mining, Ethereum selects a validator at random to propose each new block. This validator assembles pending transactions into a block, attaches the necessary information (such as the previous block’s hash), and broadcasts it to the network for verification.
Once the block is proposed, other validators on the network verify its contents, ensuring that all transactions are legitimate and that the block conforms to Ethereum’s rules. When more than 2/3rd of the validators agree, the block is added to the chain, and the validator who proposed it is rewarded with transaction fees and newly minted Ether.
Inside an Ethereum Block: Key Components
Ethereum blocks contain several critical pieces of information:
- Transaction Data: This is the main content of the block, recording all transactions included in the block. Each transaction contains details such as the sender and receiver’s addresses, the amount of Ether or token being transferred, and the gas fees paid.
- State Data: Ethereum’s unique account-based model means that each block also contains state data, which tracks the balances of every account and the status of smart contracts. This state data represents the cumulative effect of all past transactions on Ethereum, including updates to token balances and smart contract variables.
- Block Header: The block header is a compact piece of metadata summarizing the block’s key properties. It includes:
- Parent Hash: The hash of the previous block, linking the new block to its predecessor and forming the blockchain.
- State Root: A cryptographic root that summarizes the state of all accounts and contracts after the block’s transactions are applied.
- Transactions Root: A root hash summarizing all transactions in the block, ensuring their integrity.
- Receipts Root: A root that stores metadata for each transaction, like gas used and logs produced, helping to track outcomes of contract executions.
- Difficulty and Nonce (in older PoW blocks): Although now largely obsolete under PoS, these fields indicate the block's difficulty level under Ethereum’s former Proof of Work (PoW) model.
These components allow each Ethereum block to act as a self-contained record of transactions and updates to the network’s state. Every node can validate a block by checking its hashes and verifying that its transactions and state changes align with Ethereum’s rules.
In summary, blocks serve as both transaction containers and checkpoints for consensus, enabling Ethereum’s decentralized network to maintain a consistent and tamper-resistant history. By examining these blocks, any participant can verify the entire chain’s integrity and trust that Ethereum’s state is accurate and secure.
Proof of Stake: Ethereum’s Transition to Energy-Efficient Consensus
Originally, Ethereum operated on a Proof of Work (PoW) consensus model, similar to Bitcoin. This approach required miners to solve complex cryptographic puzzles to validate transactions and create new blocks. However, the energy demands of PoW, along with scalability concerns, led to a significant upgrade known as the Beacon Chain hard fork, which transitioned Ethereum to Proof of Stake (PoS) in September 2022. This shift aimed to make Ethereum more sustainable, secure, and efficient.
Understanding Proof of Stake and the Role of Validators
In Ethereum’s PoS system, participants who want to help secure the network become validators rather than miners. To join as validators, participants must "stake" a minimum of 32 ETH—a commitment that acts as a deposit, signaling that they are trusted network members.
Validators play a key role in the PoS process:
- They are responsible for proposing and validating new blocks.
- Validators also participate in the finalization process, solidifying Ethereum’s state and securing its transaction history.
If a validator is selected to propose a block, they organize pending transactions, create a new block, and broadcast it to the network. Other validators verify the new block’s contents to confirm its legitimacy, creating a secure and decentralized consensus. Coin Bureau’s guide on Ethereum Staking can help you get started with participating in the Ethereum network.
Key Metrics of Ethereum’s PoS System
Several core metrics define Ethereum’s PoS process:
- Block Time: In Ethereum’s current network, each PoS block is created approximately every 12 seconds. This interval ensures that transactions are processed efficiently and provides a steady flow of new blocks, which keeps the network responsive.
- Finality: Ethereum’s PoS model includes a concept called finality, which marks a point at which transactions are considered permanent and cannot be reversed. Finality occurs every 32 slots, a slot being a 12-second interval in which a block can be proposed. This means that every 6.4 minutes, Ethereum reaches a finalized state, solidifying the blockchain and ensuring that recent transactions are unchangeable.
- Slashing: Ethereum employs slashing to maintain security and prevent malicious behavior. Suppose a validator acts dishonestly or fails to perform their duties (such as attempting to propose conflicting blocks). In that case, they risk having a portion of their staked ETH "slashed" or permanently removed. This penalty is a deterrent, promoting honest participation and ensuring Ethereum’s cryptoeconomic security.
Liquid Staking and the Rise of LST Projects
While traditional staking requires validators to lock up their ETH, liquid staking allows stakers to retain liquidity on their assets. In liquid staking, users receive Liquid Staked Tokens (LSTs) representing their staked ETH, allowing them to use these tokens in other parts of the Ethereum ecosystem (e.g., DeFi) while their original ETH remains staked.
Although liquid staking is not a part of Ethereum’s core protocol, it has become extremely popular, with a substantial portion of all staked ETH flowing through liquid staking projects. Top liquid staking providers include:
- Lido: The largest liquid staking protocol, where users receive stETH, a tokenized version of staked ETH.
- Rocket Pool: A decentralized staking service offering rETH as a liquid token, allowing users to stake in smaller amounts or as node operators.
- Puffer finance: A newer liquid staking provider that aims to offer more accessible staking options with high-security features.
These projects offer flexibility and liquidity for stakers, making staking more accessible to the broader Ethereum community while strengthening Ethereum’s security by encouraging more staking participation.
Decentralized Applications (DApps) and DeFi
Building upon our earlier discussion of smart contracts, Decentralized Applications (DApps) operate on the Ethereum blockchain, leveraging its decentralized infrastructure to provide services without centralized control.
- Execution in the EVM: DApps run within the Ethereum Virtual Machine (EVM), Ethereum's global computational environment. The EVM processes smart contracts, ensuring that DApps execute consistently across all network nodes.
- Programmability via Smart Contracts: DApps are programmable through smart contracts written in Solidity, Ethereum's primary programming language. These contracts define the logic and rules governing the DApp's operations, enabling various functionalities.
- Turing Completeness: The EVM is Turing complete, meaning it can perform any computation given enough resources. This capability allows developers to create complex and versatile DApps. However, to prevent infinite loops and excessive resource consumption, Ethereum requires gas fees for computations. Without such a mechanism, a non-terminating process could consume infinite gas, leading to network congestion and potential abuse.
Prominent Categories of DApps in the Ethereum Ecosystem:
- Decentralized Finance (DeFi): Platforms offering financial services like lending, borrowing, and trading without intermediaries.
- Real-World Assets (RWAs): DApps that tokenize physical assets, such as real estate or commodities, enabling on-chain ownership and trading.
- Decentralized Physical Infrastructures (DePINs): Projects that decentralize physical infrastructure services, like networking or storage, through blockchain technology.
- Game Finance (GameFi): Integrates gaming with financial incentives, allowing players to earn cryptocurrency through gameplay.
- Social Finance (SocialFi): Combines social networking with financial elements, enabling users to monetize content and interactions.
- Liquid Staking: Services that allow users to stake cryptocurrencies while maintaining liquidity through tokenized representations of staked assets.
- Restaking: Mechanisms enabling users to stake assets across multiple protocols, enhancing yield opportunities.
- Yield-Bearing Stablecoins: Stablecoins that offer interest or yield, providing passive income while maintaining a stable value.
- Non-Fungible Tokens (NFTs): Platforms for creating, buying, and selling unique digital assets representing ownership of specific items or content.
- Decentralized Exchanges (DEXs): Platforms facilitating peer-to-peer cryptocurrency trading without centralized intermediaries.
Here is a list of the Top DApps in the Ethereum network.
What is Decentralized Finance (DeFi)?
Decentralized Finance (DeFi) refers to a broad ecosystem of financial applications built on blockchain networks, primarily Ethereum, that operate without traditional intermediaries like banks or brokers. DeFi aims to create an open, permissionless, and transparent financial system accessible to anyone with an internet connection.
Significance of DeFi:
- Contrast with Centralized Finance: Traditional financial systems are often characterized by centralized control, limited accessibility, and potential inefficiencies. DeFi addresses these issues by providing decentralized alternatives that are more inclusive and resistant to censorship.
- Stablecoins in DeFi: Stablecoins are cryptocurrencies pegged to stable assets, such as fiat currencies, to minimize price volatility. They are integral to the DeFi space, serving as a stable medium of exchange and a unit of account within various protocols. Stablecoins facilitate lending, borrowing, and trading activities by providing a consistent value reference.
As of November 2024, the total value locked (TVL) in Ethereum's DeFi ecosystem has reached approximately $192 billion, marking the highest level in 15 months and signaling renewed growth in the sector.
This substantial valuation underscores DeFi's expanding role in the financial landscape, offering innovative solutions and opportunities beyond the constraints of traditional finance. Check out the Best DeFi Projects by Coin Bureau.
Blockchain Explorers: Windows into the Blockchain
One of the unique features of blockchain technology is its complete transparency. Every transaction on the Ethereum network is visible to the public, creating an open ledger where all activities are recorded and verifiable by anyone. Not only are transactions transparent, but smart contract data on Ethereum is also open-source, allowing users to view the contract’s code and verify its functionality. This openness enables participants to trust the network without needing a centralized authority.
Blockchain Explorers: Browsers for On-Chain Data
To make this data easily accessible, blockchain explorers act like web browsers for the blockchain. As you use a search engine to find websites, a blockchain explorer lets you search and view on-chain data, including transaction details, account balances, and smart contract information.
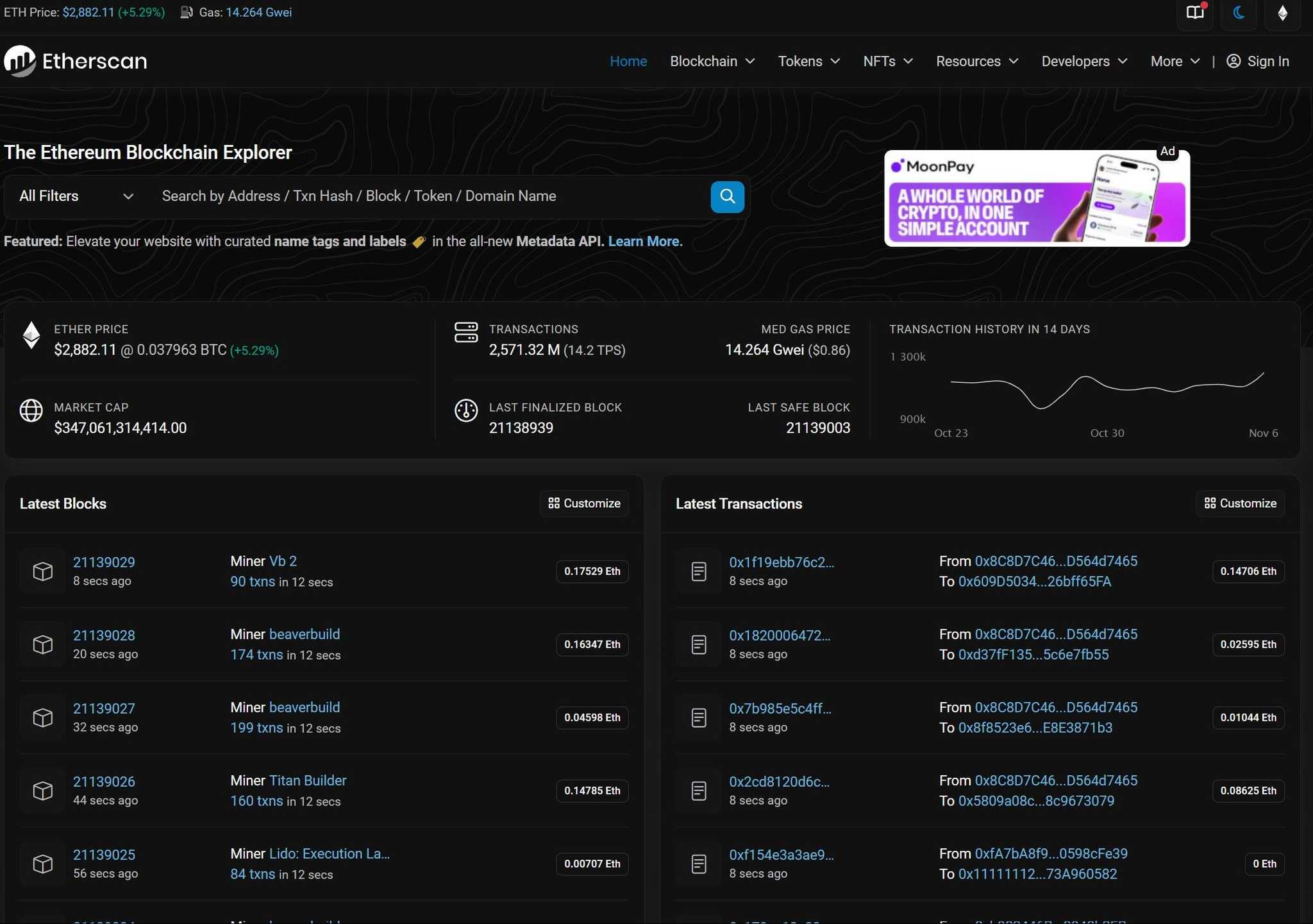
Etherscan is the most widely used blockchain explorer for Ethereum. It provides a user-friendly interface for navigating Ethereum’s blockchain, making it possible to track transactions, inspect contract details, and analyze on-chain metrics. Here’s a look at some key functions that Etherscan offers:
- Track Transaction Trails: Etherscan allows users to enter any Ethereum address or transaction hash to view the entire transaction history. You can trace the path of tokens, Ether transfers, and other activities, providing insight into how assets have moved across the network.
- Verify Transfers: Users can verify transfers of Ether and tokens, ensuring that payments or transfers have been completed successfully. Each transaction entry includes details like sender and receiver addresses, transaction amounts, and timestamps, all recorded on the blockchain.
- Audit Smart Contracts: Since Ethereum smart contracts are open-source, users can view their code directly on Etherscan. This makes it possible to audit contracts, check for potential risks, and understand the functionality of popular decentralized applications (DApps). Auditing smart contracts allows users to assess whether a contract is trustworthy before interacting.
- Check Contract Balances: Etherscan lets users check the balances of both EOAs (Externally Owned Accounts) and contract accounts. This feature helps track the holdings of decentralized applications, liquidity pools, or other DeFi projects, providing transparency about where funds are stored and how they’re managed.
- View On-Chain Metrics: Etherscan offers insights into network metrics, such as gas prices, block times, and the number of active validators. Users can also view information about network activity, including transaction volumes and trends over time. These metrics give users a deeper understanding of Ethereum’s network health and usage.
Blockchain explorers like Etherscan are essential tools for anyone interacting with Ethereum, as they offer a transparent view into the blockchain’s operations. By making all transaction and contract data easily accessible, they uphold Ethereum’s principles of openness and security, empowering users to verify and explore the network independently.
Understanding Ether Monetary Policy
Ethereum’s approach to its native currency, Ether (ETH), is unique. Ethereum is programmed with an infinite supply model, unlike Bitcoin, which has a capped supply. However, to prevent an overflow of ETH in circulation, Ethereum implemented a fundamental upgrade known as EIP-1559, which introduced a balance between new ETH entering the system and older ETH being removed.
Ethereum Blocks and Supply Control
With Ethereum’s transition to Proof of Stake (PoS), the supply of ETH is now influenced by network demand and gas fees. Validators who replace miners in PoS create blocks in response to user transaction demand. Each block adjusts the overall ETH supply depending on the gas fees users will pay and the protocol’s dynamic gas management.
What is Gas?
On Ethereum, gas measures the computational resources required to execute on-chain transactions. Every action—sending ETH, interacting with a smart contract, or deploying a new DApp—consumes gas. Gas fees are determined by the units of gas consumed in a transaction, multiplied by the current gas price (measured in gwei, where 1 gwei = 10^-9 ETH).
EIP-1559 introduced a new gas fee structure, with each transaction’s gas fee split into two components:
- Base Fee: This is the minimum gas fee required for a valid transaction. The base fee is determined by the network and fluctuates depending on transaction volume and network demand. Upon successful transaction execution, it is burned out of Ethereum’s supply.
- Priority Fee: Users can add a tip above the base fee, known as the priority fee, to incentivize validators to include their transactions in the next block. The higher the priority fee, the quicker the transaction will be processed.
Most Ethereum wallets allow users to set a gas limit, which caps the priority fee they are willing to pay. This protects users from excessive fees, as setting a gas limit prevents their transactions from endlessly consuming funds. If network demand is high, users may place a higher priority fee to avoid delays. However, if the transaction fails or is omitted, any gas spent on processing the request will not be refunded.
Ethereum Block Breakdown and Gas Management
Ethereum blocks are measured by the total gas consumed within them. Every 12 seconds, the network randomly selects a validator to propose a new block, targeting a block size of 15 million gas but allowing up to a maximum limit of 30 million gas.
To prevent transaction costs and network storage demands from soaring, Ethereum dynamically adjusts the base fee based on its proximity to the target block size:
- Target Block Size: The ideal block size is 15 million gas.
- Base Fee Adjustment: If blocks consume more than 15 million gas in two consecutive blocks, the base fee increases by 12.5% for each block. This adjustment continues until a block consumes less than 15 million gas, at which point the base fee decreases by 12.5% for each block.
EIP-1559’s gas model aims not to make gas fees cheaper but more predictable. By adjusting the base fee based on block usage, Ethereum ensures smoother pricing, reducing sudden fluctuations that previously made transaction costs volatile.
Insights into ETH Inflation and Deflation
One of EIP-1559's key impacts has been its effect on ETH supply dynamics. Each new block mints a small amount of new ETH, adding to the total supply. However, the protocol also burns the base fee—the portion of gas fees paid by users—effectively removing ETH from circulation.
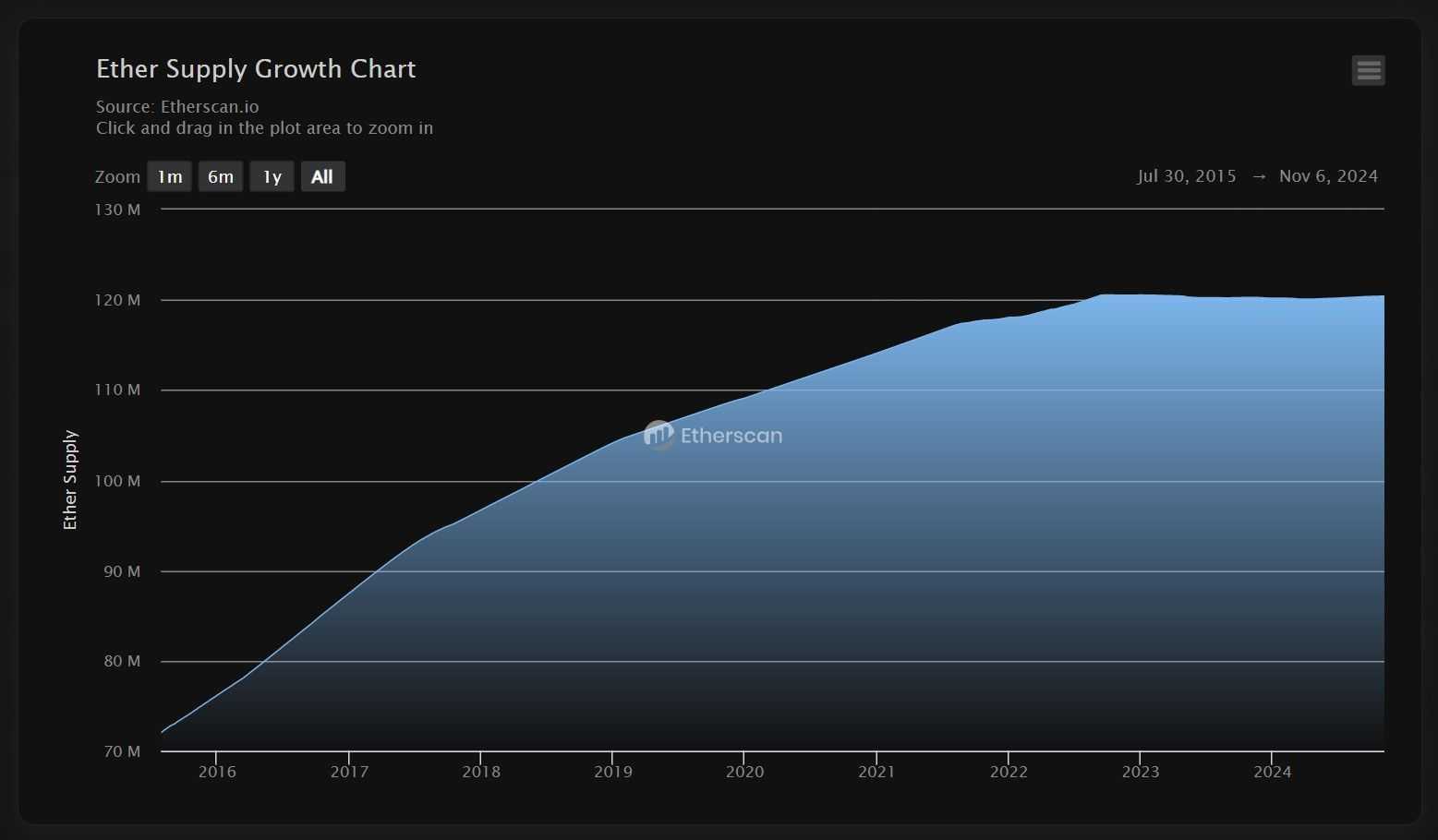
This burn mechanism has led many to describe ETH as a deflationary currency, but this is only partly accurate. Ethereum becomes deflationary only when the amount of ETH burned exceeds the amount minted, which occurs during periods of high demand when base fees spike. If demand drops, the rate of ETH burning slows, allowing the total supply to grow.
The result is a unique balance between inflation and deflation, controlled by the network’s demand and usage. During peak times, ETH supply contracts as more ETH is burned, while lower demand leads to net-positive minting. This mechanism helps keep Ethereum’s supply more balanced and responsive to market conditions.
Ethereum Blockchain Layers: The Foundation of the Ethereum Network
Ethereum’s mainnet is organized into three core layers, each serving a specific function to make the blockchain secure, functional, and decentralized. These layers—Data Availability Layer, Consensus Layer, and Execution Layer (EVM)—are essential for Ethereum’s operation. Together, they form a complete blockchain but are separated based on tasks, not physical boundaries. Each layer is contained within Ethereum’s validator nodes, creating a unified yet modular architecture.
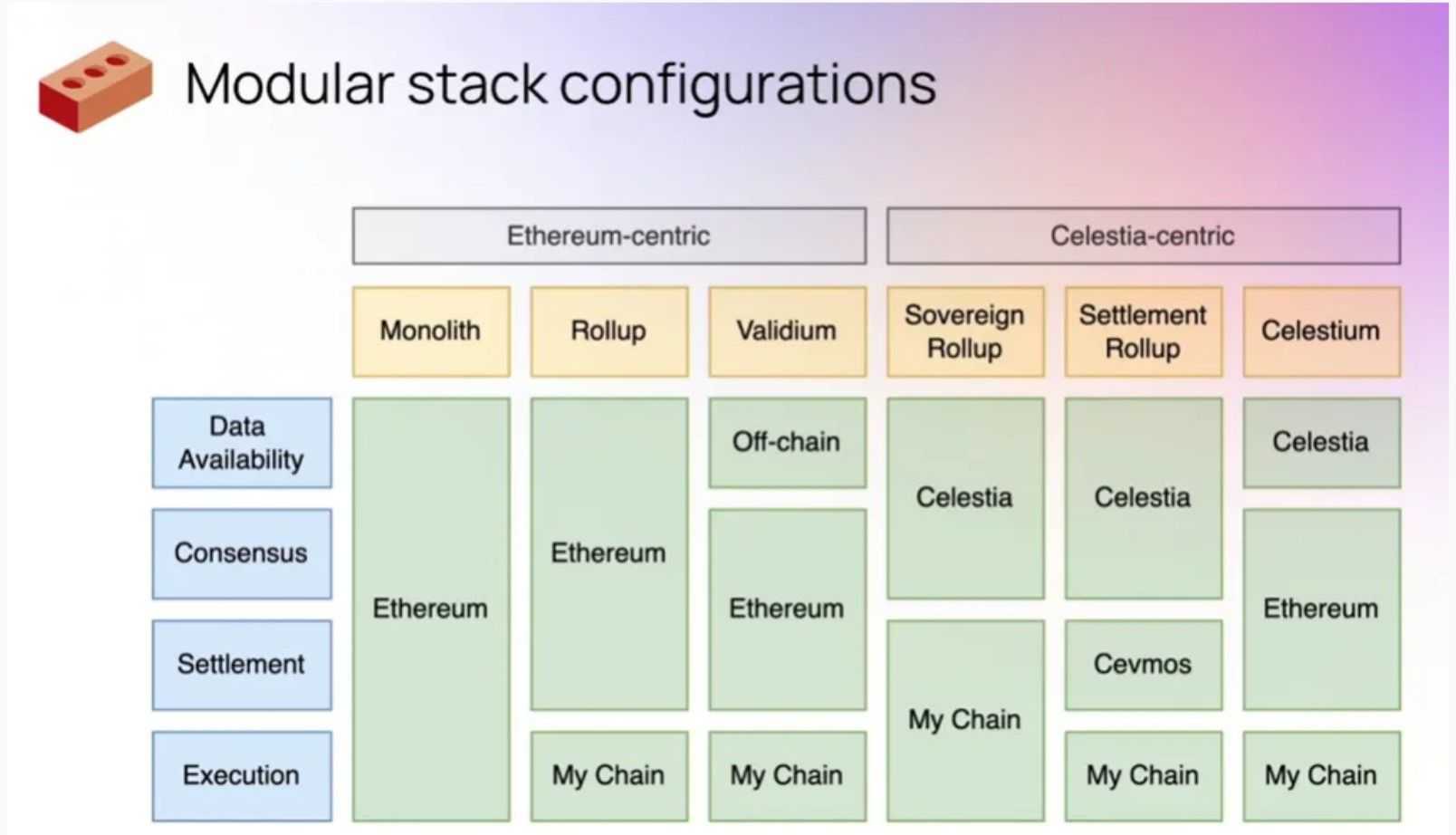
Data Availability Layer
The Data Availability Layer makes transaction data accessible to all participants. When users interact with Ethereum, every transaction and smart contract interaction generates data that must be broadcast to the network. This layer ensures that this data is available to validators and nodes, allowing them to verify transactions and synchronize Ethereum’s state.
Data availability is critical because it guarantees that all nodes see the same data, maintaining transparency and preventing data from being withheld or hidden by malicious actors. This layer is key to preserving the network’s liveness and ensuring that every validator can independently verify the chain’s state.
Consensus Layer
The Consensus Layer is the backbone of Ethereum’s security. It coordinates Ethereum’s Proof of Stake (PoS) consensus mechanism, where validators agree on the current state of the blockchain. The layer is responsible for validating transactions, adding new blocks, and ensuring all nodes follow the same rules.
Through this layer, Ethereum achieves consensus on legitimate transactions and finalized, making it resistant to double-spending attacks and network manipulation. The Consensus Layer keeps Ethereum decentralized, as validators work together to maintain and secure the chain.
Execution Layer (EVM)
The Execution Layer, also known as the Ethereum Virtual Machine (EVM), is where the actual execution of smart contracts and transactions occurs. This layer processes computations, manages smart contract logic, and updates account balances. Think of it as Ethereum’s computational engine, handling everything from token transfers to DeFi protocols.
The EVM allows Ethereum to function as a programmable blockchain capable of running decentralized applications (DApps) and complex contract logic. By separating execution from data availability and consensus, Ethereum can focus on each task efficiently without overwhelming the network.
How These Layers Create the Ethereum Mainnet
Together, these layers combine to form the Ethereum mainnet. They exist within each validator node, providing distinct functionalities while remaining interconnected within its architecture. This separation of duties makes the Ethereum network more resilient, as each layer independently manages a core blockchain function, allowing validators to maintain a consistent and verified ledger across the network.
The Scalability Trilemma
Like most blockchains, Ethereum faces the scalability trilemma—the challenge of simultaneously achieving scalability, security, and decentralization. When all tasks of the blockchain layers (data availability, consensus, and execution) are performed by the same network of nodes, they create a bottleneck that restricts scalability. Here’s how each pillar of the trilemma is affected:
- Security: Ethereum maintains strong security standards by prioritizing the consensus layer. However, increasing network security often involves sacrificing speed.
- Decentralization: Decentralization requires validators to share responsibilities, preventing one party from controlling the network. However, with all layers performing tasks together on each node, it becomes difficult to scale without sacrificing some level of decentralization.
- Scalability: For Ethereum to process more transactions quickly, the network needs to increase throughput. However, with all layers combined, higher transaction volumes put pressure on each layer, especially the Execution Layer, leading to slower processing times.
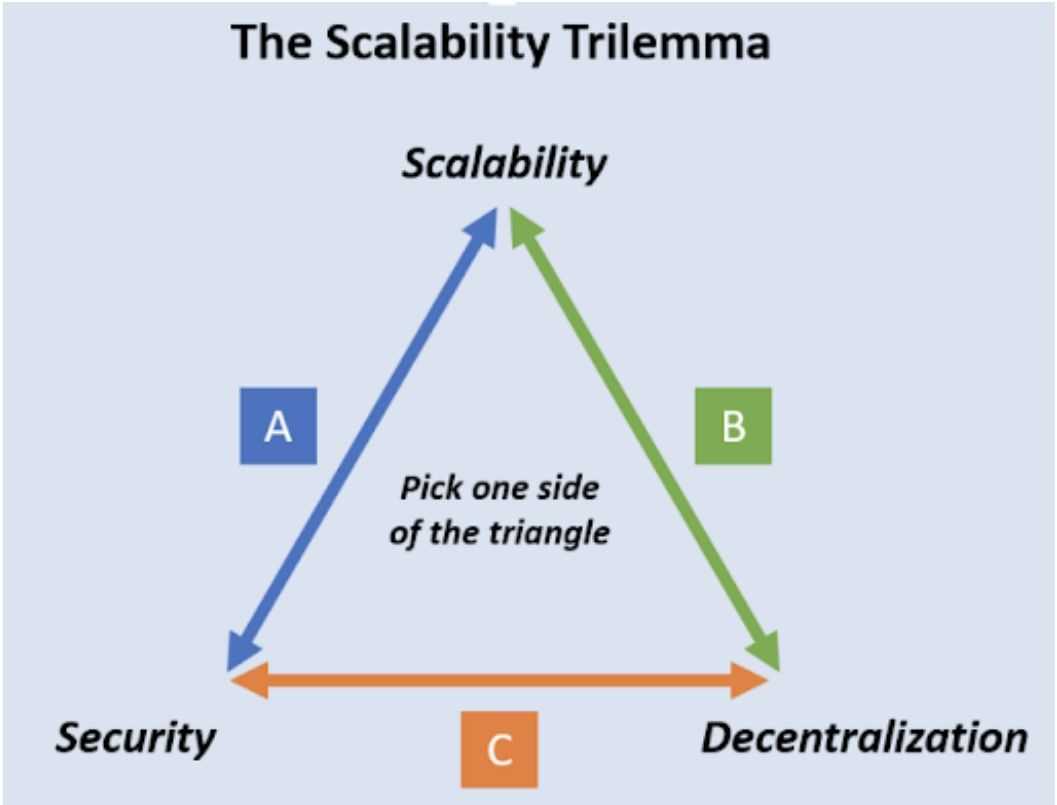
Ethereum’s trilemma lies in balancing these three goals, as any emphasis on one can strain the others. The need to scale without compromising security or decentralization led Ethereum to adopt a Rollup-centric roadmap.
Rollup-Centric Roadmap: Ethereum’s Solution to the Trilemma
To overcome the trilemma, Ethereum adopted a rollup-centric roadmap that offloads scalability functions to a new layer while maintaining a secure and decentralized mainnet.
- Focus on Security and Decentralization: Under this roadmap, Ethereum’s mainnet is optimized to handle Data Availability and Consensus layers, while the Execution Layer is partially offloaded to a separate layer. By focusing on security and decentralization, Ethereum can maintain the integrity of the network while achieving greater scalability through external solutions.
- Introduction of Layer 2 Solutions: This roadmap led to developing Layer 2 (L2) solutions, or “rollups.” Layer 2 protocols are specialized execution environments that handle many transactions outside the main Ethereum chain but periodically report back to the Ethereum mainnet. Rollups handle execution off-chain, then bundle and submit the transaction data to Ethereum’s mainnet for security and finalization.
This rollup-centric approach addresses Ethereum’s scalability issues by distributing the workload. The mainnet ensures data availability and consensus, while Layer 2 ecosystems provide efficient transaction processing. As Layer 2 networks continue to develop, Ethereum’s ecosystem has become more capable of handling high demand while maintaining its security and decentralization.
Scaling the Ethereum Ecosystem: The Rollup-Centric Roadmap
In the previous section, we discussed the different layers of the Ethereum mainnet and introduced the idea of a rollup-centric roadmap. Let's dive deeper into this topic to understand Ethereum's evolution and how it addresses scalability challenges.
Blockchain Modularity: Splitting the Chain for Higher Scalability
Blockchain modularity involves separating a blockchain's core functions—Data Availability (DA), consensus, and execution—into distinct layers. By modularizing these components, Ethereum aims to achieve higher scalability while maintaining a cohesive ecosystem. Each layer specializes in its specific task:
- Data Availability Layer: Ensures that transaction data is accessible to all network participants.
- Consensus Layer: Maintains network security by verifying and agreeing on the state of the blockchain.
- Execution Layer (EVM): Handles the computation and execution of smart contracts.
The Ethereum community recognized that scaling the ecosystem without losing security and decentralization was only possible by modularizing Ethereum. By delegating specific functions to dedicated layers, the network can handle increased demand more efficiently without overloading any single component.
Ethereum's Core Strength: Source of Credible Block Space
As the Ethereum ecosystem grew, it became the most decentralized blockchain network due to its extensive network of validators. Ethereum's core strength isn't just that most applications and DeFi projects are built on it; rather, it offers the most secure blockchain network through its Data Availability layer upheld by validators.
What is Credible Block Space?
Credible block space refers to the secure and reliable space on the blockchain where transactions and data are stored and verified. In the context of Ethereum, this credible block space is underpinned by the Data Availability layer. Ethereum's validators collectively facilitate the most secure block space in Web3.
What makes Ethereum's block space particularly valuable is its arbitrary usability. This means the block space isn't limited to securing only Ethereum transactions; other networks can rent it to secure their networks as sandboxed environments within Ethereum. This "sandboxed environment" analogy illustrates how other protocols can leverage Ethereum's robust security without interfering with the mainnet's operations.
By offering credible block space, Ethereum provides a foundation for other projects to build securely, benefiting from the network's decentralization and consensus mechanisms.
What is the Rollup-Centric Roadmap?
Building on these insights, the rollup-centric roadmap represents Ethereum's evolved focus on making its credible block space more scalable and accessible. The roadmap outlines a strategy where Ethereum's mainnet specializes in the Data Availability and Consensus layers. In contrast, the Execution layer is shifted to a new layer handled by Layer 2 solutions, known as rollups.
Rollups are scaling solutions that process transactions off the main Ethereum chain but return transaction data to the mainnet for security and consensus. By doing so, they:
- Increase Scalability: Offloading execution to rollups reduces congestion on the mainnet, allowing for higher transaction throughput.
- Maintain Security and Decentralization: Since rollups rely on Ethereum's credible block space for security, they inherit the mainnet's robust security features.
- Enhance Accessibility: Rollups make it more affordable and efficient for users to interact with the Ethereum ecosystem by lowering gas fees and increasing transaction speeds.
The rollup-centric roadmap allows Ethereum to focus on its strengths—providing secure and decentralized block space—while rollups handle scalability. This division of labor enables Ethereum to grow sustainably without compromising its foundational principles.
In the next section, I will explain how credible block space motivated the rise of the Ethereum Layer 2 ecosystem.
The Ethereum Layer 2 Ecosystem
Ethereum's Layer 2 solutions (or L2s) are separate blockchain networks that work alongside Ethereum to execute transactions efficiently while leveraging Ethereum for security and data availability. These L2s provide a scalable framework that allows high transaction throughput, lower costs, and enhanced user experience, all while inheriting Ethereum's security and decentralization.
How Ethereum Layer 2s Work
An Ethereum Layer 2 is designed as an independent blockchain network that processes large transactions off the main Ethereum chain. However, instead of functioning independently, it relies on Ethereum’s Data Availability (DA) and Consensus layers for security and finality. Here’s how the process works:
- Sequencer Nodes: Layer 2s uses a specialized node called a sequencer to collect, validate, and execute transactions in high volumes. This sequencer node plays a crucial role by ordering and bundling transactions into large batches, optimizing throughput, and reducing congestion.
- Batching Transactions and Validity Proofs: Once the sequencer processes a batch of transactions, it constructs a combined validity proof that represents the integrity of the entire batch. Some Layer 2s, known as zk-rollups, utilize zero-knowledge proofs (ZK proofs) to create a succinct validity proofs. ZK proofs allow these rollups to confirm that the transactions in a batch are valid without revealing detailed information about each transaction, which enhances privacy and efficiency.
- Posting Batches to Ethereum: After the sequencer creates the validity proof, the rollup posts the bundled transactions and the proof on Ethereum as a single transaction. This transaction is handled by a rollup smart contract deployed on Ethereum, which verifies the validity of the batch based on the proof submitted.
- Leveraging Ethereum’s Credible Block Space: Once posted, the Ethereum DA layer stores copies of the rollup’s data across the network, effectively vouching for the rollup by guaranteeing data availability. This design allows the rollup to leverage Ethereum’s credible block space, ensuring that even if Layer 2 encounters issues, the data remains accessible on Ethereum, and users retain the ability to recover their transactions.
- Cost Efficiency and Security: By batching multiple transactions into a single proof, the cost of posting data to Ethereum is divided across all transactions within the batch. This dramatically reduces the cost per transaction, allowing Layer 2s to offer low fees while inheriting Ethereum’s security, liveness, and decentralization.
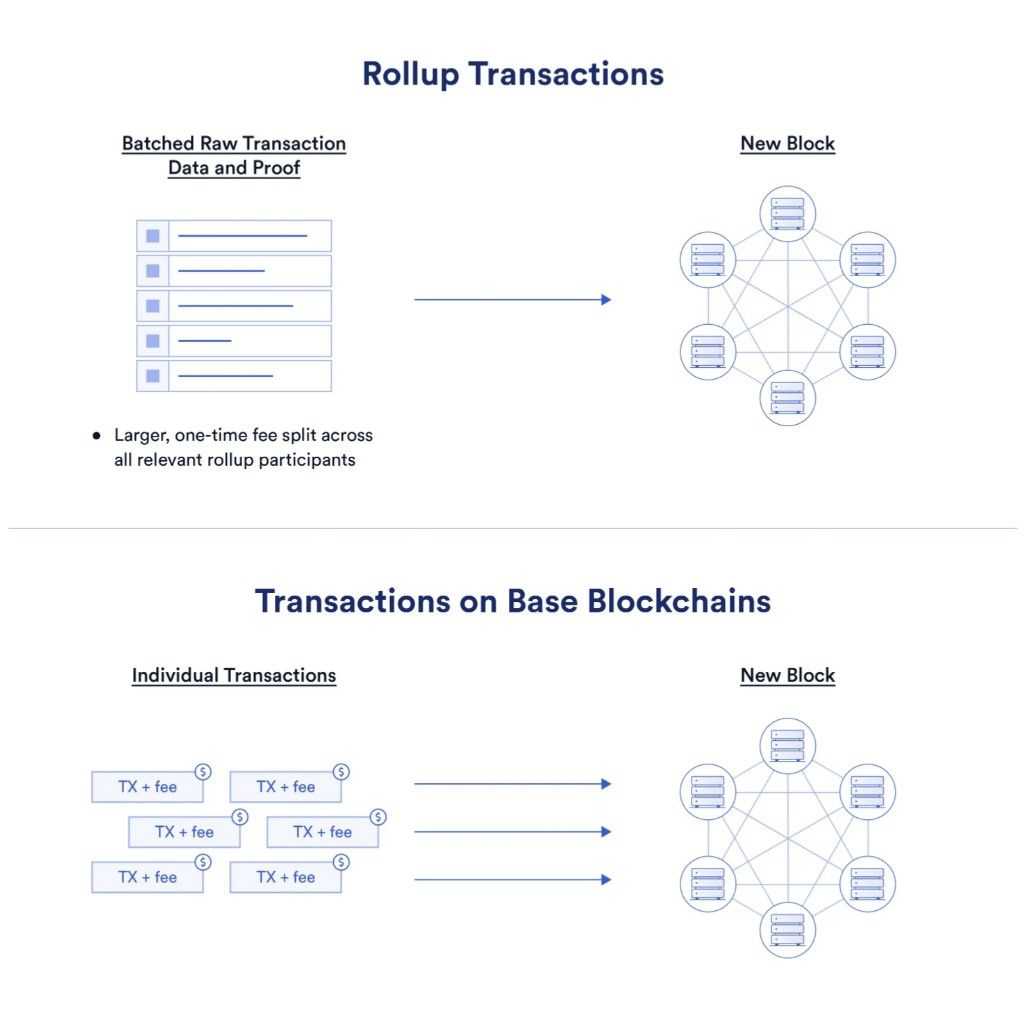
In summary, Ethereum Layer 2s, like zk-rollups enhance scalability by offloading transaction execution while relying on Ethereum to secure the network’s data and integrity. Ethereum can scale sustainably through this symbiotic relationship without sacrificing security or decentralization.
Proto-Danksharding: Making Credible Block Space Cheaper
In March 2024, Ethereum implemented the Dencun upgrade, which introduced EIP-4844, known as Proto-Danksharding. This significant enhancement reduced the cost of credible block space for Layer 2 solutions by nearly 90%, fostering greater scalability and efficiency within the Ethereum ecosystem.
Understanding the Upgrade Through a Computer Analogy
To grasp the impact of this upgrade, let's revisit our earlier analogy of Ethereum as a computer:
- Before the Upgrade: Layer 2 solutions stored their transaction data in Ethereum's RAM. In computing, RAM is where active processing occurs; it's limited in capacity and relatively expensive. In Ethereum, this corresponds to CALLDATA, the space where transaction data is processed and stored. Storing large amounts of data in CALLDATA was costly and constrained by space limitations.
- After the Upgrade: With the Dencun upgrade, Layer 2 solutions stored their data in Ethereum's ROM. ROM is used for storage that doesn't require constant processing, offering larger capacity and lower costs. In Ethereum, this new storage method is called BLOB DATA (Binary Large Objects Data). By shifting data storage from CALLDATA to BLOB DATA, Layer 2s can store information more efficiently and affordably.
Introducing Blob Data and Blob Gas
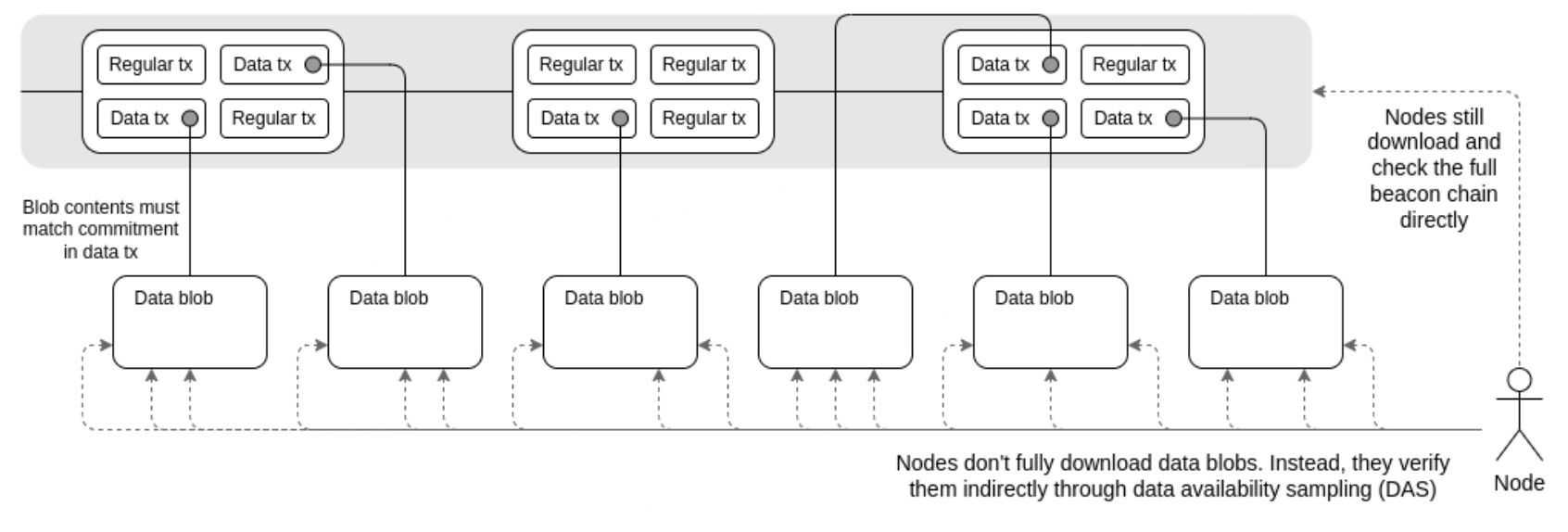
The Dencun upgrade provided a new storage format and introduced a separate gas mechanism called Blob Gas. This system operates parallel to the existing gas mechanism, maintaining a similar structure but at a significantly lower cost. By utilizing Blob Gas, BLOB DATA transactions become more economical, reducing the overall expenses for Layer 2 operations.
Impact on the Ethereum Ecosystem
By enabling BLOB DATA and Blob Gas, Ethereum has made its credible block space more accessible and affordable. This advancement promotes the growth of the Layer 2 ecosystem, allowing for higher transaction throughput and lower fees. Consequently, Ethereum has solidified its position as the largest network of interconnected chains in Web3, offering a scalable and secure platform for decentralized applications.
For a deeper understanding of Ethereum Layer 2 solutions, readers can explore more resources on Coin Bureau.
The Ethereum Roadmap: History and Future of Upgrades
Ethereum’s evolution is guided by a series of community-driven proposals known as Ethereum Improvement Proposals (EIPs). EIPs serve as a formalized process for proposing, discussing, and implementing changes to the Ethereum network. They range from small technical adjustments to major network upgrades and are essential for refining Ethereum’s functionality, scalability, and security.
What Are EIPs?
An EIP is a document that proposes a new feature, process, or improvement to the Ethereum network. Each EIP outlines the motivation behind the proposed change, the technical details, and the expected impact on the network. EIPs follow a structured lifecycle, including community discussions, testing, and final approval or rejection. Once accepted, an EIP may be incorporated into a future upgrade, helping Ethereum adapt to new challenges and improve its overall efficiency.
The Process Behind Ethereum Upgrades
The Ethereum community has established a rough system for researching and developing upgrades:
- Idea and Draft Stage: Developers, researchers, or community members propose new ideas based on observed needs, industry trends, or innovative research. These ideas are often debated within the community to evaluate their potential impact.
- EIP Proposal: If an idea gains support, it moves to the formal EIP stage, where it is documented in detail. The proposal explains the problem being addressed, outlines the solution, and includes technical specifications.
- Review and Discussion: The Ethereum community, including core developers and stakeholders, reviews the EIP. This open process allows for feedback, improvements, and modifications based on community input.
- Testing and Development: Promising EIPs enter the testing phase. Here, developers simulate the proposed changes on test networks to evaluate their effectiveness, check for bugs, and ensure compatibility with existing features.
- Incorporation into Network Upgrades: If testing is successful, the EIP is scheduled for inclusion in an upcoming Ethereum upgrade. These upgrades, often named after cities or concepts (e.g., “Dencun” or “Shanghai”), are planned to introduce multiple EIPs in a coordinated release, gradually enhancing the network.
This structured process ensures that Ethereum remains a collaborative, community-driven project, with EIPs forming the basis for continuous improvement. In the following sections, we’ll explore specific upgrades in the Ethereum roadmap that are set to advance the network significantly. Here are some of the foundational EIPs passed in the network:
- Frontier - July 30, 2015: This was the initial release of the Ethereum network, a barebones implementation intended for developers.
- Frontier Thawing – September 7, 2015: This update lifted the gas limit per block, allowing for transactions to be processed and preparing for future upgrades.
- Homestead – March 14, 2016: This upgrade introduced protocol and networking changes, enhancing the network's capabilities and paving the way for future upgrades.
- DAO Fork – July 20, 2016: In response to a hack of The DAO, a decentralized autonomous organization, this fork moved funds from the compromised contract to a new one, allowing users to withdraw their ETH.
- Tangerine Whistle – October 18, 2016: This fork addressed network health issues related to underpriced operation codes, mitigating denial-of-service attacks.
- Spurious Dragon – November 22, 2016: A further response to DoS attacks, this upgrade tuned opcode pricing, enabled blockchain state "debloat," and added replay attack protection.
- Byzantium – October 16, 2017: This upgrade reduced block rewards, delayed the "difficulty bomb," and added features like non-state-changing calls and cryptography methods for layer 2 scaling.
- Constantinople – February 28, 2019: This upgrade reduced block rewards, ensured the network's continued operation, optimized gas costs, and introduced the ability to interact with uncreated addresses.
- Istanbul – December 8, 2019: This upgrade optimized gas costs, improved resilience against DoS attacks, enhanced the performance of layer 2 solutions, enabled interoperability with Zcash, and expanded contract functionality.
- Muir Glacier – January 2, 2020: This upgrade delayed the "difficulty bomb," preventing degradation of network usability by mitigating increasing block difficulty.
- Staking Deposit Contract Deployed – October 14, 2020: This introduced staking to Ethereum, paving the way for the Beacon Chain launch.
- Beacon Chain Genesis - December 1, 2020: This marked the launch of the Beacon Chain, a significant step toward Ethereum's Proof-of-Stake vision.
- Berlin – April 15, 2020: This upgrade optimized gas costs and increased support for various transaction types.
- London – August 5, 2021: This upgrade introduced EIP-1559, reforming the transaction fee market for greater predictability and efficiency.
- Altair – October 27, 2021: This upgrade to the Beacon Chain added support for "sync committees," facilitating light clients, and increased validator penalties.
- Arrow Glacier – December 9, 2021: This upgrade pushed back the "difficulty bomb," ensuring smooth network operation.
- Gray Glacier – June 30, 2022: Similar to Arrow Glacier, this upgrade further delayed the "difficulty bomb.”
- Bellatrix – September 6, 2022: This upgrade prepared the Beacon Chain for The Merge, adjusting validator penalties and fork choice rules.
- Paris (The Merge) - September 15, 2022: This monumental upgrade transitioned Ethereum from Proof-of-Work to Proof-of-Stake, marking a significant milestone in its history.
- Shanghai-Capella ("Shapella") – April 12, 2023: This upgrade enabled staking withdrawals from the Beacon Chain to the execution layer, a crucial feature for stakers.
- Cancun-Deneb ("Dencun") – March 13, 2024: This upgrade focuses on scalability, introducing EIP-4844 (Proto-Danksharding) to reduce data storage costs for layer 2 rollups, resulting in lower transaction fees for users.
Here are some of the most anticipated EIPs still under research in the Ethereum community:
Account Abstraction: Simplifying Ethereum User Interactions
One anticipated upgrade in Ethereum’s roadmap is Account Abstraction, a concept designed to make user interactions on Ethereum more flexible, efficient, and user-friendly. Traditionally, Ethereum has two types of accounts: externally owned accounts (EOAs) controlled by private keys and Contract Accounts governed by code. Account abstraction aims to blur this distinction, enabling all accounts to operate more like smart contracts and allowing custom transaction logic.
Account Abstraction essentially lets users customize their accounts by introducing programmable features, such as multi-signature requirements, social recovery mechanisms, and meta-transactions. This flexibility is useful for onboarding users unfamiliar with private key management, making Ethereum more accessible and secure.
Key EIPs Under Account Abstraction
Two primary EIPs contribute to Ethereum’s account abstraction: EIP-4337 and EIP-7702. Let’s look at each in detail.
EIP-4337: Decentralized Account Abstraction Without Consensus Changes
EIP-4337 proposes a system for implementing account abstraction without changing Ethereum’s core consensus. This is achieved by introducing a new type of "User Operation" that operates independently of the standard Ethereum transaction model.
Here’s how it works:
- User Operations: EIP-4337 introduces a concept called "User Operations," which is a way for users to interact with Ethereum without relying on traditional private key transactions. Instead, these operations are handled by a separate pool called the "User Operation Mempool."
- Bundlers: Special nodes, known as bundlers, aggregate multiple User Operations and submit them as a single transaction to the Ethereum network. This allows for more efficient processing of transactions, as users can batch multiple operations into one.
- Smart Contract Wallets: EIP-4337 enables all accounts to function as smart contract wallets, meaning users can include additional logic, such as daily spending limits or multi-sig requirements. This approach enhances security and user control, making Ethereum accounts more versatile.
The major benefit of EIP-4337 is that it allows for account abstraction without altering Ethereum’s underlying consensus mechanism. This makes it easier to implement while still achieving the benefits of customizable accounts and flexible transaction models.
EIP-7702: Temporary Smart Contract Code for EOAs
EIP-7702, co-authored by Vitalik Buterin, proposes a new transaction type that allows Externally Owned Accounts (EOAs) to temporarily set a smart contract code executable only during the transaction. This approach enables EOAs to access account abstraction features without permanently migrating to smart contract accounts or delegating transaction control to external contracts.
Key aspects of EIP-7702 include:
- Temporary Contract Code: EOAs can include a contract_code field in their transactions, specifying code that is active only for the duration of that transaction.
- Enhanced Functionality: This mechanism allows EOAs to perform complex operations such as gas sponsorships, batch transactions, and custom execution logic without altering their fundamental structure.
- Alignment with Account Abstraction Roadmap: By enabling temporary smart contract capabilities, EIP-7702 aligns with Ethereum's broader goal of unifying account functionalities while preserving the simplicity of EOAs.
Together, EIP-4337 and EIP-7702 represent significant steps toward a more flexible and user-friendly Ethereum ecosystem, allowing for enhanced account functionalities without compromising the network's security or requiring extensive structural changes.
Proposer-Builder Separation (PBS): Enhancing Ethereum’s Security and Scalability
Proposer-Builder Separation (PBS) is an upcoming upgrade that aims to improve Ethereum’s security and scalability by dividing the core responsibilities of validators. Currently, validators are responsible for building and verifying blocks, creating potential inefficiencies and security risks. With PBS, these roles will be split between two specialized participants: block builders and proposers (Ethereum validators). PBS optimizes block space usage by separating block construction and verification and addresses security concerns such as Maximal Extractable Value (MEV).
What is Maximal Extractable Value (MEV), and Why is it a Security Concern?
Maximal Extractable Value (MEV) refers to the maximum profit a validator can make by reordering, including, or excluding transactions within a block. MEV arises because specific transaction placements can be exploited to generate profit, incentivizing validators to manipulate transaction orders.
A typical example of MEV exploitation is the sandwich attack, often seen in decentralized exchanges (DEXs). In a sandwich attack, a validator detects a large trade about to happen and performs the following actions:
- The validator places a trade right before the large trade to drive up the asset's price.
- The large trade executes, further raising the asset’s price.
- The validator then sells the asset immediately after the large trade, profiting from the increased price.
This process extracts value from other users and compromises network security and fairness. PBS aims to address MEV by introducing a more transparent and regulated approach.
How PBS Works
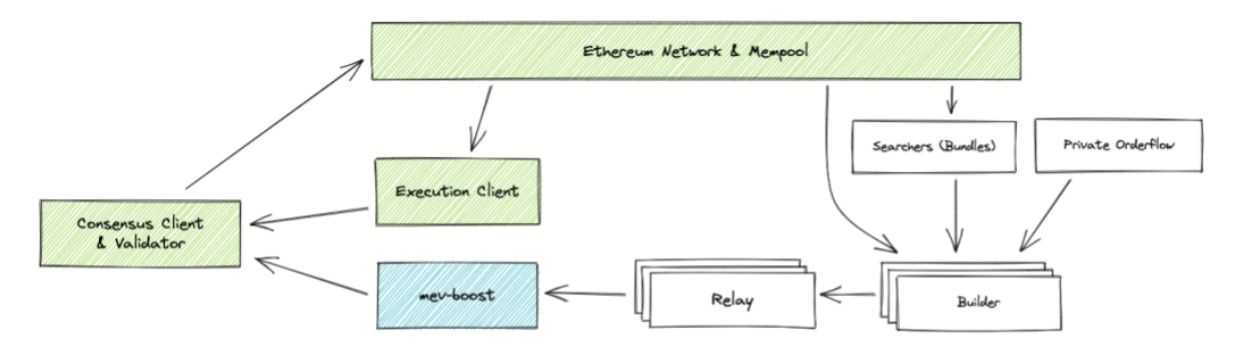
PBS will introduce a new network participant called Block Builders, specializes in constructing the most efficient blocks. Here’s a breakdown of the PBS process:
- Block Building by Block Builders: Block builders collect transactions and use specialized algorithms to create efficient blocks that maximize network throughput while accounting for MEV. They produce blocks that are optimized for the highest profitability without compromising integrity.
- Block Auction: Once block builders have created the blocks, they enter an auction where proposers (Ethereum validators) select the block with the highest bid.
- Block Verification by Proposers: The proposers verify the transactions within the chosen block, ensuring they meet Ethereum’s standards. If the block is valid, it is accepted by the network. The winning bid is distributed among the participating builders and proposers, creating an incentive structure that rewards efficient block production.
How PBS Enhances Ethereum’s Security and Scalability
- Efficient Block Space Usage: With block builders optimizing the construction of each block, Ethereum’s block space is used more effectively, increasing the number of transactions processed per block and thus enhancing scalability.
- Transparent MEV: PBS makes MEV public, integrating it into Ethereum’s consensus mechanism. This transparency allows MEV practices to be monitored and controlled, reducing the risk of malicious activities and enabling the Ethereum protocol to punish exploitative MEV tactics.
- Reduced Risk of Collusion and Bias: Under the current system, validators handle both block construction and verification, allowing the potential for biased behavior or collusion. PBS eliminates this risk by separating these duties, with proposers focused solely on verification. This ensures a neutral block selection process and reduces opportunities for collusion.
PBS is still under active research and development within the Ethereum community as developers continue to refine its design to maximize security and scalability benefits.
Danksharding: Ethereum's Vision for Full Scalability
Danksharding represents the complete realization of Ethereum's scalability roadmap, building on the foundational work of Proto-Danksharding. While Proto-Danksharding introduced blob-carrying transactions—allowing rollups to store data efficiently within each block—Danksharding takes this concept to the next level, further enhancing the network’s capacity to support high-throughput applications and reduce Layer 2 (L2) transaction costs.
From Proto-Danksharding to Danksharding
Proto-Danksharding, already live on the Ethereum mainnet, allows each Ethereum block to carry up to 6 blobs of data. These blobs are data sections dedicated to rollups, enabling high-volume transaction processing without congesting the Ethereum mainnet. Blobs make data available but do not require every Ethereum node to execute the transactions, reducing computational strain.
If Proto-Danksharding proves successful, full Danksharding will increase the blob limit per block from 6 to 64, dramatically multiplying the network's capacity for rollup data and reducing transaction costs for Layer 2 solutions. With more blobs per block, Ethereum can handle even greater volumes of transactions off-chain, further reducing congestion and gas fees on the mainnet.
Managing Increased Storage Requirements
As promising as Danksharding is, increasing the blob limit per block introduces significant storage requirements for Ethereum nodes. Higher blob capacity means more data stored in each block, potentially making it challenging for validators to maintain a full copy of the blockchain without substantial increases in storage.
One proposed solution to address this challenge is to prune blob data older than one month from the mainnet. This means that blob data would be removed from actively stored data on nodes after one month, freeing up space and making storage demands more manageable.
This solution is viable because:
- Finalized Data: Blob data older than one month is finalized and immutable, meaning it has been thoroughly validated and can no longer be altered.
- Minimal Risk of Forks or Inconsistencies: After one month, the chances of network forks or inconsistencies affecting the integrity of old blob data are extremely low, making pruning a safe and effective way to reduce storage overhead.
Danksharding thus offers Ethereum a scalable future, leveraging optimized data management to support the network’s growth and cementing Ethereum as a leader in Web3 scalability.
Pectra: The Ethereum Meta EIP
The Ethereum Pectra Upgrade is a significant forthcoming enhancement to the Ethereum network aimed at improving scalability, efficiency, and user experience. Scheduled to roll out in two phases starting in early 2025, Pectra combines two previously planned upgrades—Prague and Electra—into a cohesive update.
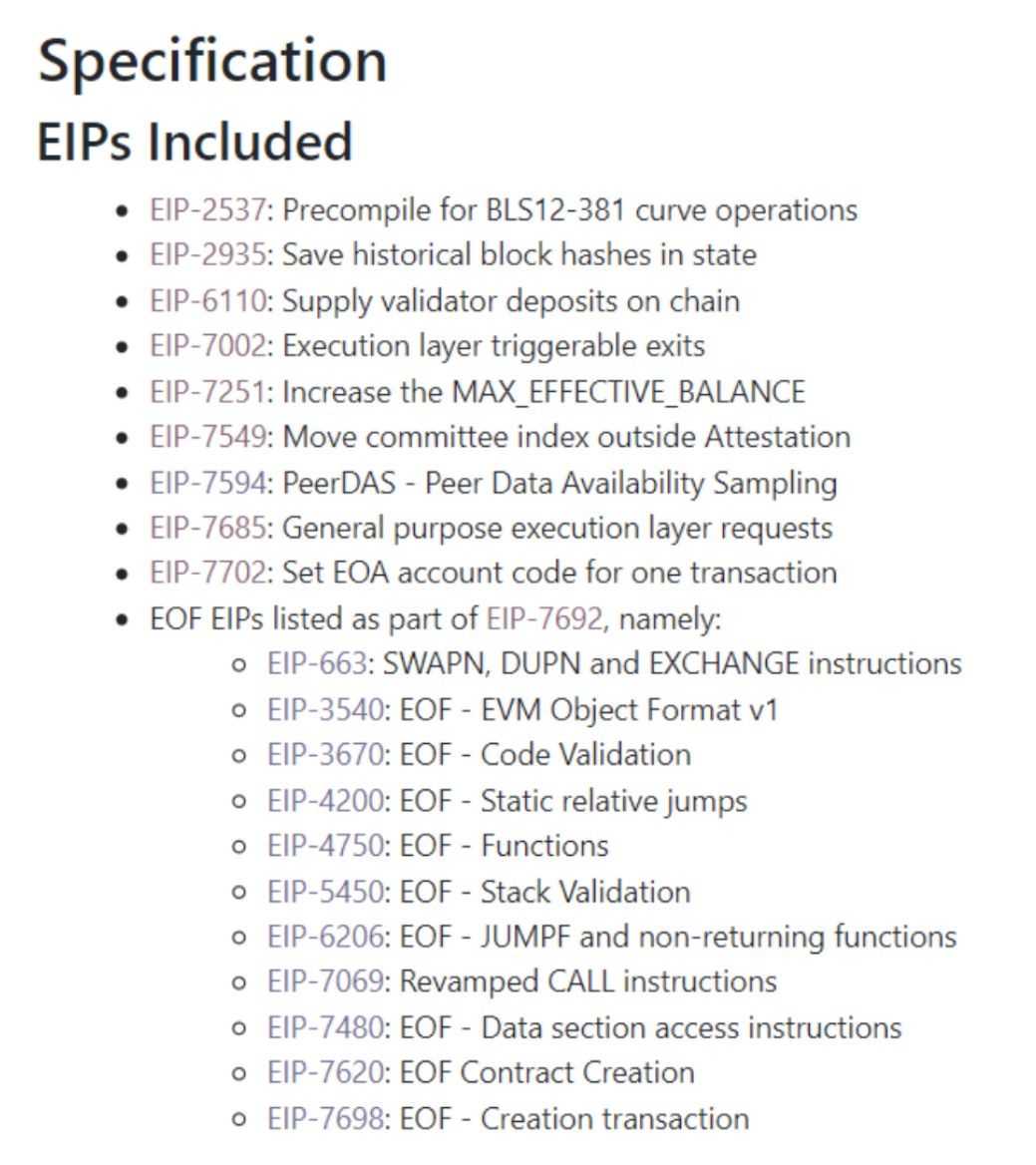
Key Features of the Pectra Upgrade:
- Account Abstraction: Pectra introduces account abstraction, allowing users to pay gas fees with various tokens, not just Ether (ETH). This flexibility enhances user convenience and broadens the usability of the Ethereum network.
- Smart Contract Efficiency: The upgrade includes improvements to the Ethereum Virtual Machine (EVM), making smart contract execution more efficient. This enhancement reduces costs and complexity for developers and users interacting with decentralized applications (DApps).
- Validator Staking Enhancements: Pectra increases the maximum stake for validators from 32 ETH to 2,048 ETH, allowing for more flexible staking options. This change aims to optimize network security and efficiency by accommodating larger staking operations.
- Introduction of Verkle Trees: The upgrade implements Verkle trees, a data structure that improves data storage and verification processes. This advancement enhances the network's scalability by reducing the amount of data nodes need to store, leading to faster transaction processing and lower costs.
- Support for Layer 2 Solutions and PeerDAS: Pectra enhances Layer 2 solutions by introducing Peer Data Availability Sampling (PeerDAS), a technology that facilitates data handling for these networks. This improvement supports Ethereum's scalability by enabling more efficient off-chain transaction processing.
By integrating these features, the Pectra Upgrade aims to make Ethereum more accessible, efficient, and scalable, benefiting both everyday users and developers within the ecosystem.
What is Ethereum: Closing Thoughts
In revisiting Ethereum’s ecosystem, we gain a new appreciation for how vast and dynamic this network has become. Over the years, Ethereum has evolved from a single-layer blockchain into a complex ecosystem designed to accommodate the broadest range of applications, assets, and users.
What’s particularly striking is the irony of Ethereum’s architecture. Ethereum has become the most intricate, deeply researched, and widely adopted blockchain network, yet its underlying ethos is to remain a generalized platform. While other Layer 1 blockchain, like Solana with its focus on scalability or Cosmos with its emphasis on interoperability, are purpose-built, Ethereum is designed to be the most versatile and general-purpose solution. This adaptability has positioned Ethereum as the central platform for many innovations, making it the most comprehensive blockchain in scope.
Moreover, this revisited exploration underscores that we can no longer discuss Ethereum as a singular network. Today, Ethereum is much closer to embodying the structure of the Internet itself—a vast, interconnected network of networks, encompassing diverse assets, applications, and communities. Ethereum has grown to be more than just a blockchain; it is a platform that underpins the broader Web3 ecosystem, reflecting its continued expansion and profound influence on the digital world.
Frequently Asked Questions
Ethereum is a decentralized blockchain platform that functions as a global computer. It allows users to run decentralized applications (DApps) and execute smart contracts, which are self-executing contracts with the terms of the agreement directly written into code. Unlike Bitcoin, which primarily focuses on peer-to-peer transfers of value, Ethereum offers a versatile ecosystem for developers to build and deploy applications without intermediaries.
While Bitcoin focuses on being a store of value and a peer-to-peer payment system, Ethereum is designed to be a flexible platform for decentralized applications. Bitcoin’s blockchain is optimized for recording BTC transactions, while Ethereum’s network supports complex computations, decentralized finance (DeFi), non-fungible tokens (NFTs), and more. Ethereum also transitioned to a Proof of Stake (PoS) consensus mechanism, making it more energy-efficient than Bitcoin’s Proof of Work (PoW) system.
Ether (ETH) is the native cryptocurrency of the Ethereum network. It is used to pay for transaction fees (gas), compensate validators who secure the network, and serve as a medium of exchange within the ecosystem. Unlike Bitcoin, which has a fixed supply, Ethereum has an infinite supply but employs mechanisms like fee burning to balance inflation.
Layer 2 (L2) solutions are independent networks that run on top of Ethereum to increase scalability and reduce transaction costs. These solutions, like zk-rollups and optimistic rollups, offload transaction execution to a secondary layer while leveraging Ethereum’s mainnet for security and data availability. L2 solutions allow Ethereum to handle higher transaction volumes more efficiently.
EIP-1559, implemented in August 2021, reformed Ethereum’s fee structure by introducing a base fee that is burned, reducing the total supply of ETH. This mechanism has a deflationary effect when network activity is high, helping to balance inflation by burning a portion of gas fees.
Proto-Danksharding, introduced in March 2024, reduces the cost of storing data on Ethereum by implementing a new type of transaction called blob-carrying transactions. This upgrade makes Layer 2 rollups more efficient, lowering transaction fees and increasing scalability.
Account abstraction aims to make Ethereum accounts more flexible by allowing users to customize transaction logic, such as enabling social recovery or gas payments with tokens other than ETH. EIP-4337 introduces "User Operations" to achieve this without changing Ethereum’s core consensus, making wallets and accounts more versatile.
Ethereum’s future involves scaling through Layer 2 solutions, implementing full Danksharding, and introducing upgrades like account abstraction and proposer-builder separation (PBS). These enhancements aim to make Ethereum more scalable, secure, and user-friendly while reducing transaction costs and increasing throughput.
Yes, Ethereum is highly secure due to its decentralized validator network and cryptographic mechanisms. However, like any blockchain, smart contracts deployed on Ethereum can contain vulnerabilities if not properly coded or audited. Users are advised to exercise caution when interacting with new projects and DApps.
Disclaimer: These are the writer’s opinions and should not be considered investment advice. Readers should do their own research.